T. J. Ballinger1, S. Bigalke2, J. E. Walsh1,3, B. Brettschneider4, R. L. Thoman1,3, U. S. Bhatt5, E. Hanna6, I. Hanssen-Bauer7, S. -J. Kim8, J. E. Overland9, and M. Wang9,10
1International Arctic Research Center, University of Alaska Fairbanks, Fairbanks, AK, USA
2Department of Geography, Portland State University, Portland, OR, USA
3Alaska Center for Climate Assessment and Policy, University of Alaska Fairbanks, Fairbanks, AK, USA
4National Weather Service Alaska Region, NOAA, Anchorage, AK, USA
5Geophysical Institute, University of Alaska Fairbanks, Fairbanks, AK, USA
6Department of Geography and Lincoln Climate Research Group, University of Lincoln, Lincoln, UK
7Norwegian Meteorological Institute, Oslo, Norway
8Korea Polar Research Institute, Incheon, Republic of Korea
9Pacific Marine Environmental Laboratory, NOAA, Seattle, WA, USA
10Cooperative Institute for Climate, Ocean, and Ecosystem Studies, University of Washington, Seattle, WA, USA
Headlines
- Above-average pan-Arctic (60-90° N) annual surface air temperatures continued in 2023, ranking 6th warmest since 1900.
- The Barents Sea region exhibited above-average surface air temperatures in all seasons, punctuated by large anomalies in autumn 2022 and winter 2023.
- The warmest summer (July-September mean) on record was observed in 2023.
Introduction
Warming near-surface air and upper-ocean temperatures in the Arctic represent an ongoing signature of Arctic change (Ballinger et al. 2022). This regional warming rate exceeds that of the global mean temperature, a phenomenon known as Arctic Amplification (e.g., Serreze and Barry 2011). Air temperature warming is associated with changes in the Arctic hydrologic cycle, including increased seasonal precipitation totals and short-term extremes (see essay Precipitation), as well as declines in terrestrial snow, land ice, and sea ice coverage (Box et al. 2021; see essays Terrestrial Snow Cover, Greenland Ice Sheet, and Sea Ice). The increase in Arctic air temperatures is congruent with more frequent extreme air temperature events, which can have detrimental, rapid, and direct biophysical impacts within the Arctic (e.g., thaw-driven coastal erosion; Walsh et al. 2020) with indirect effects that permeate globally (e.g., as land ice mass losses contribute to sea-level rise; Moon et al. 2019). Thus, Arctic warming has far-reaching long-term consequences beyond the region.
Consistent with previous Arctic Report Card Surface Air Temperature essays, we provide the historical context of this past year’s Arctic (60-90° N) air temperatures followed by a seasonal synopsis of air temperature patterns.
Arctic annual air temperatures
Long-term Arctic and Global surface air temperature anomalies from NASA’s GISTEMP (version 4) and the ERA5 reanalysis (see Methods and data for details) are shown for their respective full periods of record in Fig. 1 following the water year (i.e., October-September mean). Considering the GISTEMP and ERA5 records, the 2023 annual anomaly was 0.76°C and 0.77°C, respectively, for the Arctic, ranking as the 6th warmest since 1900. For the GISTEMP record, this year marks the 14th consecutive year where Arctic temperatures have exceeded the 1991-2020 mean, while the eight warmest years in the Arctic have all occurred since 2016. Within this past year, autumn, winter, and spring Arctic air temperatures saw >90th percentile warmth with summer air temperatures emerging as the record warmest in over 120 years.
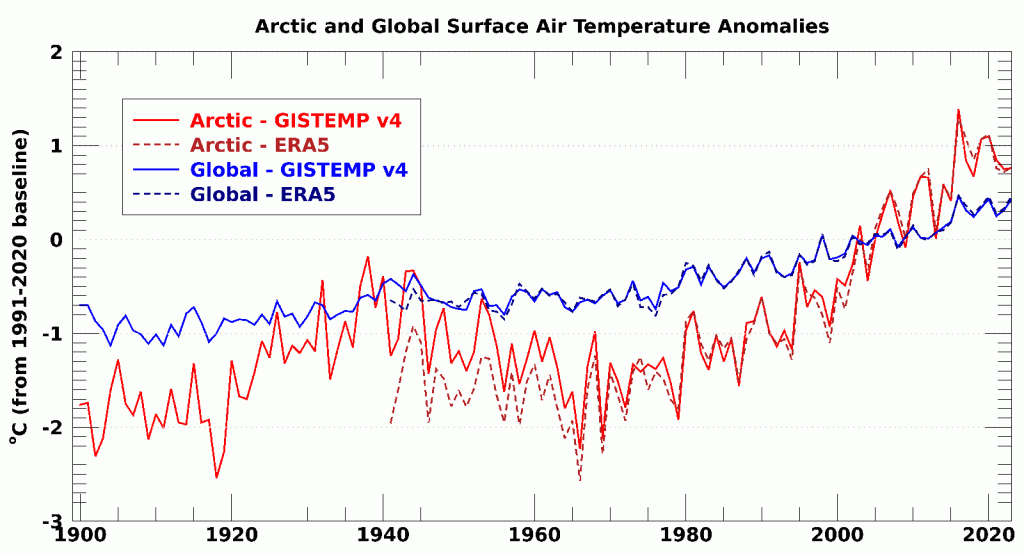
The Arctic Amplification signal is clear and persistent at the annual scale as shown by warmer Arctic surface air temperature anomalies relative to those measured for the whole of planet Earth (Fig. 1). Diverse, complex, and interrelated mechanisms and feedbacks underlie accelerated Arctic warming. For example, less extensive and thinner sea ice tends (see essay Sea Ice) to melt out earlier in the year. Longer periods of open water and sparse ice during summer result in more direct and prolonged transfer of atmospheric energy into the Arctic Ocean. As a result, the upper-ocean cools more slowly, delaying sea ice formation as upper-ocean heat is released to the atmosphere, warming the surface air temperatures in autumn and early winter (Serreze and Barry 2011). In today’s Arctic, these interactions affect the marginal ice zones and adjacent coastal areas, most notably in the Chukchi and Beaufort Seas (Ballinger et al. 2023) and Barents Sea (Isaksen et al. 2022). These examples are further touched upon in the following section.
Seasonal air temperature patterns
Arctic surface air temperature anomaly patterns are further discussed at the seasonal scale as follows: autumn 2022 (October-December [OND]), winter (January-March [JFM]), spring (April-June [AMJ]), and summer (July-September [JAS]) 2023 (Fig. 2). The seasons are defined to span the water year (October-September) and coincide with annual cycles of variables consistently referenced in Arctic Report Card essays. For example, Arctic sea-ice melt begins in spring, while late summer (i.e., September) tends to mark the annual sea ice cover minimum.
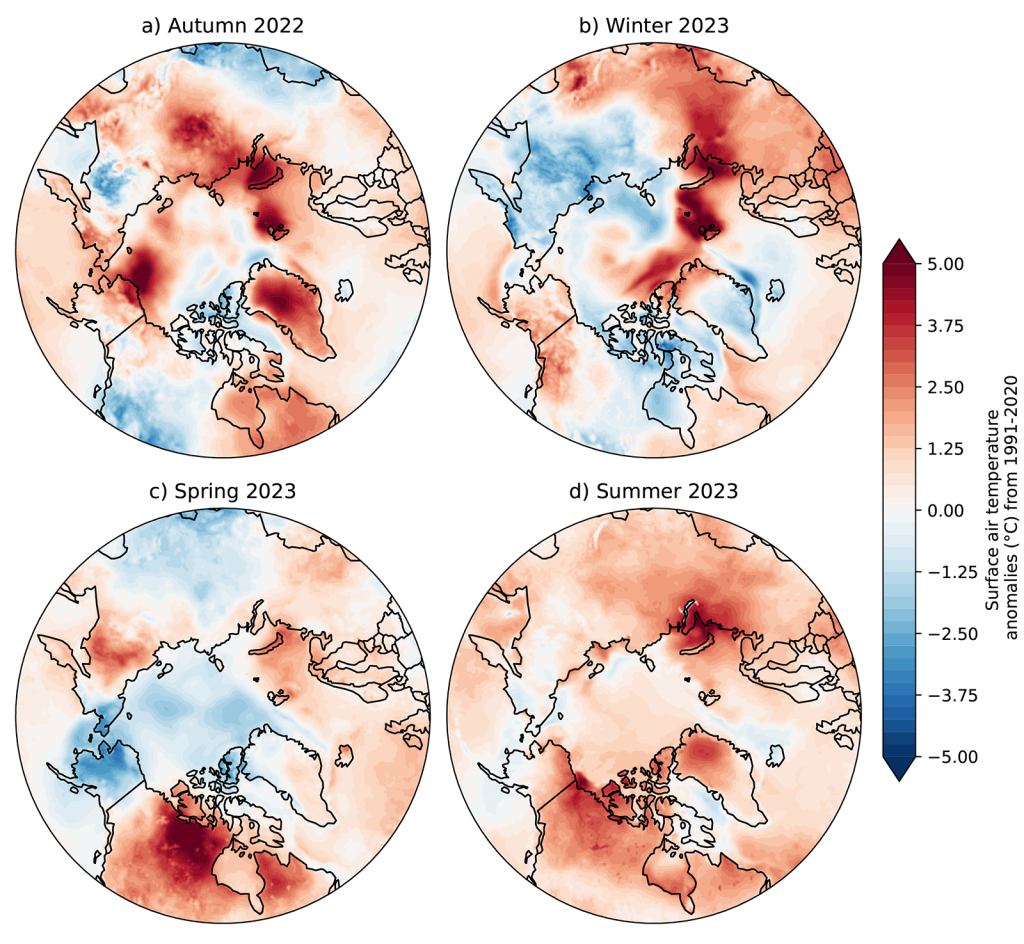
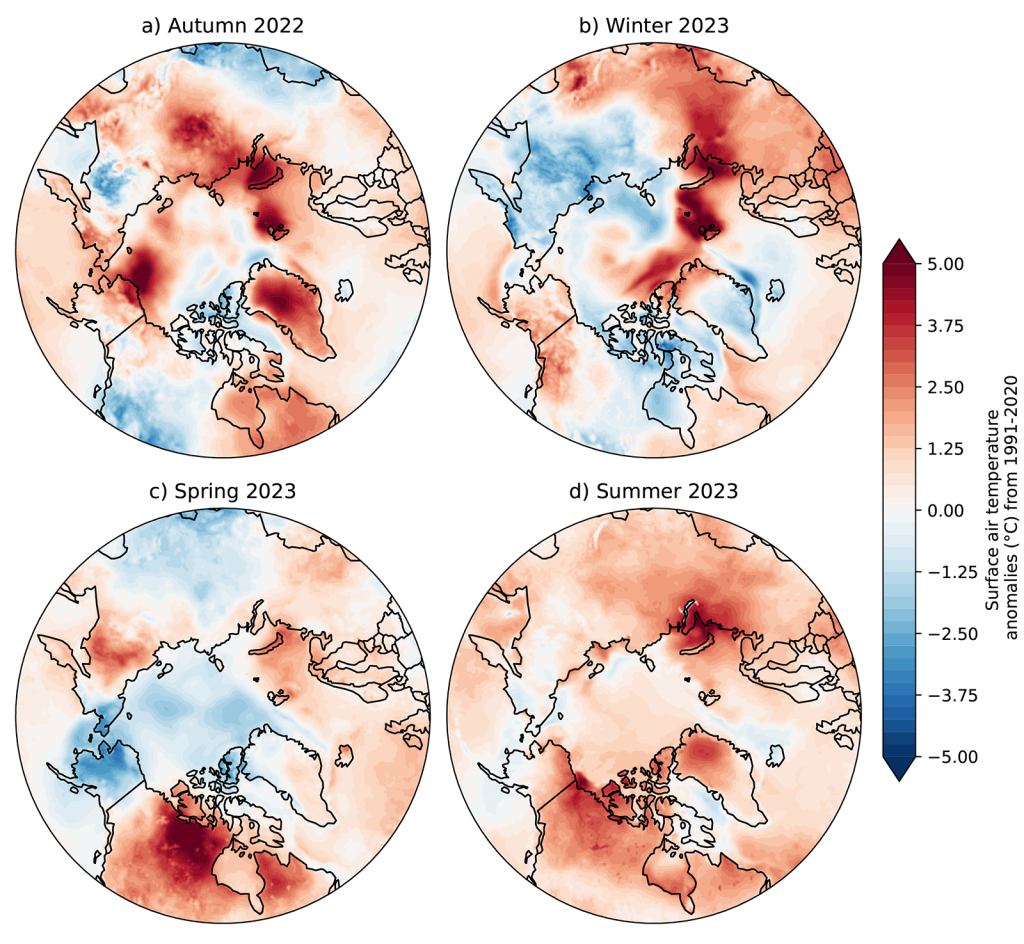
During autumn 2022, the largest warm anomalies (~5°C) were found over the Arctic marginal seas and nearby coastal zones, including eastern Chukchi and western Beaufort Seas and North Slope of Alaska, the interior of the Greenland Ice Sheet, atop Svalbard, and over the westernmost Barents Sea (Fig. 2a). Of note, a high temperature extreme at Utqiaġvik on 5 December 2022 (4.4°C) set a daily maximum temperature record for any date between 30 October and 22 April (Rick Thoman, personal communication). Warm anomalies were also apparent over north-central Eurasia, Chukotka, and northern Quebec. A negative sea-level pressure anomaly over the Laptev and East Siberian Seas transported warm air poleward into the Pacific Arctic from the Eurasian continent (Fig. 3a). The summer sea ice loss and delayed ice formation in autumn (see essay Sea Ice), combined with the large-scale atmospheric pressure pattern, may have contributed to the warm anomalies over Chukotka and the Chukchi and Beaufort areas.
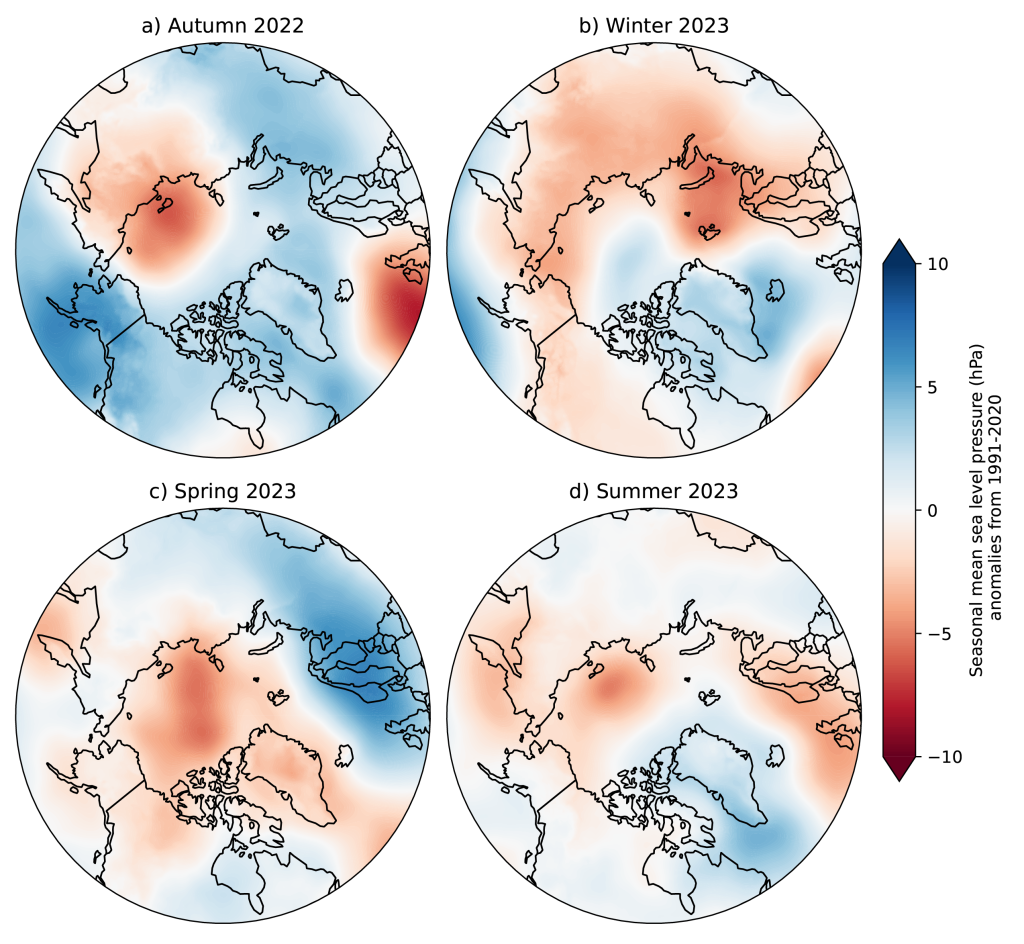
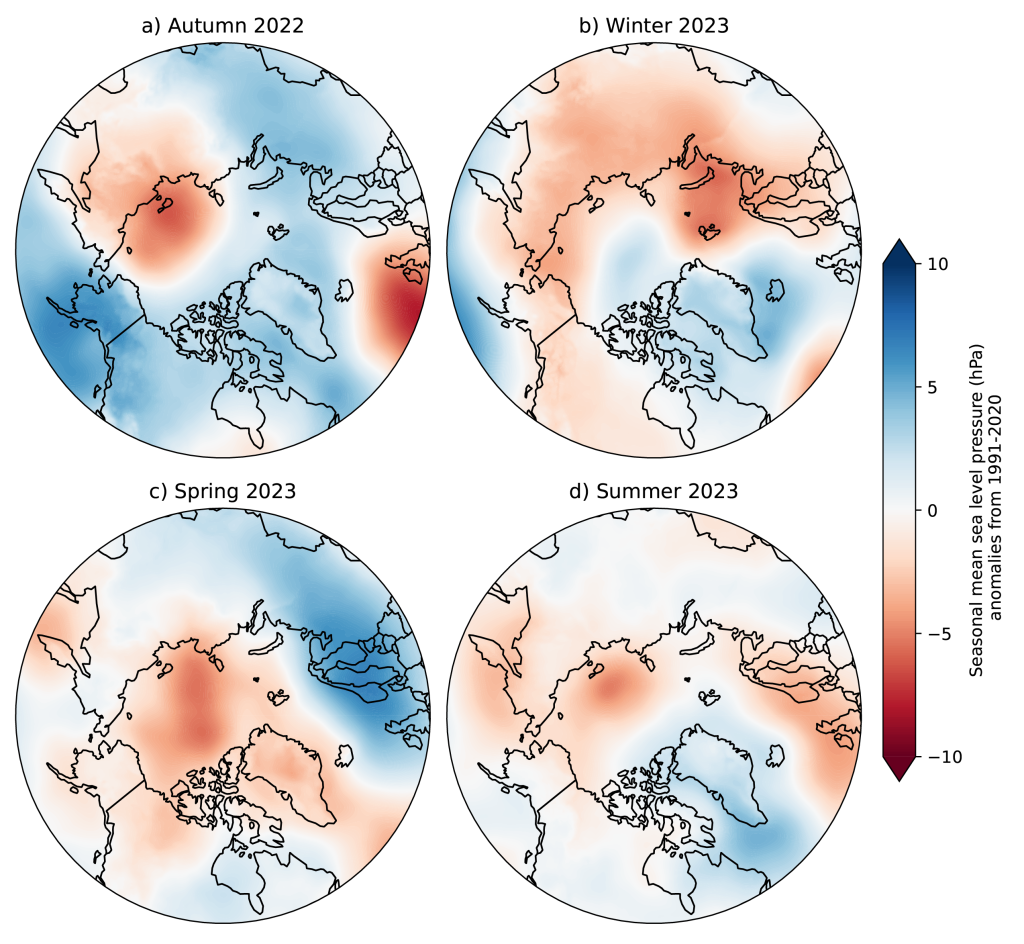
The warmest anomalies in winter 2023 were noted over the northern and southern Barents Sea and northwestern Eurasia (~5°C) (Fig. 2b). Additional positive temperature anomalies are shown over the Lincoln Sea and just north of the Canadian Arctic Archipelago. Much of central and eastern Siberia, Hudson Bay, the southern Canadian Arctic Archipelago, and Greenland Sea were characterized by cold anomalies. Negative surface pressure anomalies were found across much of the Arctic landscape and over the Barents Sea (Fig. 3b), suggestive of an active high-latitude winter storm track linked with the observed mild temperatures.
Spring 2023 showed notable warming over the Northwest Territories and Nunavut (~5°C anomalies; Fig. 2c) associated with reduced snow cover and a shorter snow cover duration (see essay Snow Cover). The north Atlantic Arctic, from central Greenland stretching to the Barents Sea and eastern Eurasia, also exhibited warmer-than-average conditions. In contrast, below-average air temperatures (~-2-3°C) were found across Alaska, the northern Bering and southern Chukchi Seas, and Chukotka. Much of the Arctic Ocean air temperatures were also near or slightly below average, associated with a low-pressure anomaly across the Central Arctic Ocean (Fig 3c).
Summer 2023, the warmest captured by NASA GISTEMP v4 since at least 1900 and ERA5 since 1941, was punctuated by warm air temperatures over most of the northern Canadian provincial areas and southern Barents Sea and Kara Sea (Fig. 2d). Regionally, the anomalous warmth atop the Northwest Territories, Nunavut, and the Canadian Arctic Archipelago coincided with below-normal precipitation over these areas (see essay Precipitation) and contributed to Northern Canada’s extreme wildfire season (see Arctic Wildfire Sidebar). Warm anomalies in northern and central Greenland were associated with near-record cumulative melt-day area across the ice sheet (see essay Greenland Ice Sheet). Warm air temperatures were also found in conjunction with low pressure anomaly patterns atop much of northern Europe, over the Laptev Sea, western Bering Sea, Kamchatka, and Sea of Okhotsk (Fig. 3d).
Methods and data
The NASA Goddard Institute for Space Studies surface temperature analysis version 4 (GISTEMP v4) is used to describe long-term Arctic (60-90° N) and Global (90° S-90° N) surface air temperatures since 1900 (Fig. 1). GISTEMP v4 air temperatures over lands are obtained from the NOAA Global Historical Climatology Network version 4 (GHCN v4) dataset and ocean surface temperatures are taken from the NOAA Extended Reconstructed Sea Surface Temperature version 5 (ERSST v5) dataset. The creation of the GISTEMP product is described in Hansen et al. (2010) and Lenssen et al. (2019).
We supplement the GISTEMP v4 data with an Arctic two-meter (i.e., surface) air temperature time series from ERA5 reanalysis (Hersbach et al. 2020). Comparison of these respective Arctic and Global time series shows good agreement with minimal differences during the last four decades (Fig. 1). We use both surface air temperature and sea-level pressure fields from ERA5 in Figs. 2 and 3, respectively. All values and fields are presented as anomalies with respect to the 1991-2020 mean.
References
Ballinger, T. J., and Coauthors, 2022: Surface air temperature. Arctic Report Card 2021, M. L. Druckenmiller, R. L. Thoman, and T. A. Moon, Eds., https://doi.org/10.25923/13qm-2576.
Ballinger, T. J., and Coauthors, 2023: Alaska terrestrial and marine climate trends, 1957-2021. J. Climate, 36, 4375-4391, https://doi.org/10.1175/JCLI-D-22-0434.1.
Box, J. E., and Coauthors, 2021: Recent developments in Arctic climate observational indicators. AMAP Arctic Climate Change Update 2021: Key Trends and Impacts. Arctic Monitoring and Assessment Programme (AMAP), Tromso, Norway, pp. 7-29.
Hansen, J., R. Ruedy, M. Sato, and K. Lo, 2010: Global surface temperature change. Rev. Geophys., 48, RG4004, https://doi.org/10.1029/2010RG000345.
Hersbach, H., and Coauthors, 2020: The ERA5 global reanalysis. Quart. J. Roy. Meteor. Soc., 146, 1999-2049, https://doi.org/10.1002/qj.3803.
Isaksen, K., and Coauthors, 2022: Exceptional warming over the Barents area. Sci. Rep., 12, 9371, https://doi.org/10.1038/s41598-022-13568-5.
Lenssen, N. J. L., G. A. Schmidt, J. E. Hansen, M. J. Menne, A. Persin, R. Ruedy, and D. Zyss, 2019: Improvements in the GISTEMP uncertainty model. J. Geophys. Res.-Atmos., 124, 6307-6326, https://doi.org/10.1029/2018JD029522.
Moon, T. A., and Coauthors, 2019: The expanding footprint of rapid Arctic change. Earth’s Future, 7, 212-218, https://doi.org/10.1029/2018EF001088.
Serreze, M. C., and R. G. Barry, 2011: Processes and impacts of Arctic amplification: A research synthesis. Global Planet. Change, 77, 85-96, https://doi.org/10.1016/j.gloplacha.2011.03.004.
Walsh, J. E., T. J. Ballinger, E. S. Euskirchen, E. Hanna, J. Mård, J. E. Overland, H. Tangen, and T. Vihma, 2020: Extreme weather and climate events in northern areas: A review. Earth-Sci. Rev., 209, 103324, https://doi.org/10.1016/j.earscirev.2020.103324.
November 17, 2023