J. K. Willis1 and M. Wood1,2
1Jet Propulsion Laboratory, California Institute of Technology, Pasadena, CA, USA
2Moss Landing Marine Laboratories, San José State University, San José, CA, USA
Highlights
- From 2015 through 2021, NASA’s Oceans Melting Greenland (OMG) experiment measured pan-Greenland changes in near-coast ice elevation, ocean temperature and salinity, and the near-shore ocean bathymetry that connects them.
- OMG showed that ice loss through glaciers at the ice sheet’s margin is strongly affected by ocean temperatures on the continental shelf.
- Argo-like profiling floats offer a robust, easily deployed, and economical tool to continue monitoring Greenland’s shelf waters.
Introduction
Greenland’s ice sheet is disappearing. Each year, the snow added in the winter falls short of replenishing the summer melt and ice loss through Greenland’s marine-terminating glaciers (see essay Greenland Ice Sheet). Because so many of Greenland’s glaciers reach the ocean and sit in hundreds of meters of water (Morlighem et al. 2017), they can be directly influenced by ocean conditions. But how much of Greenland’s ice loss is driven by the oceans? It was this question that inspired NASA’s 6-year long airborne mission called Oceans Melting Greenland—OMG, for short.
While it was already known that some glaciers could be strongly influenced by ocean conditions (Holland et al. 2008; Straneo and Heimbach 2013), OMG set out to determine how widespread this influence could be and to quantify it. During its mission, OMG collected comprehensive observations of ocean depth on the shelf and near many glacier termini, ice elevation change measurements of all marine-terminating glaciers where they met the ocean, and widespread observations of ocean temperature and salinity on the continental shelf around the entire ice sheet (Fig. 1). Armed with this extensive set of new observations, OMG showed Greenland’s glaciers are extremely sensitive to changes in ocean temperature. In the process, OMG also discovered new techniques for measuring ocean conditions in key locations on Greenland’s continental shelf.
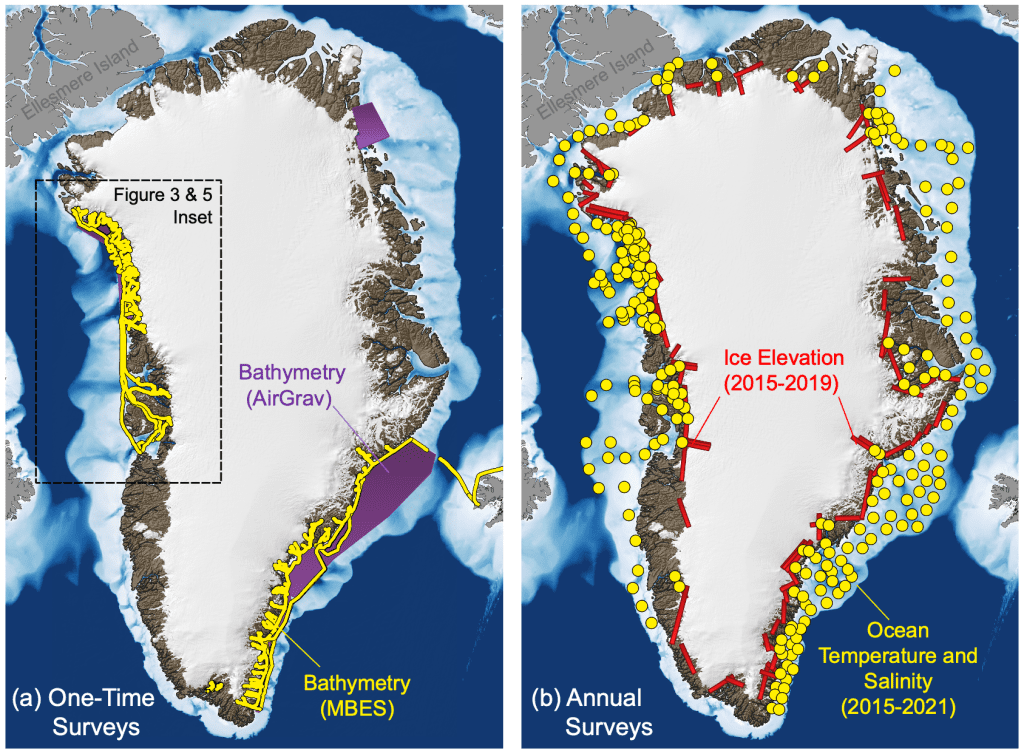
Pervasive Atlantic water and its variability
All around Greenland, warm, salty water from the Atlantic Ocean lies beneath a layer of colder, fresher water that comes from the Arctic (Fig. 2). Because this warm water sits deeper than 100 to 200 m below the ocean surface, it is impossible to use remote sensing techniques to directly observe these temperatures, and even inferred estimates face complex challenges and depend strongly on local oceanographic conditions (Snow et al. 2021). This means that direct, in situ observations of waters on the shelf are critically important for explaining ongoing ice loss and projecting future sea level rise.
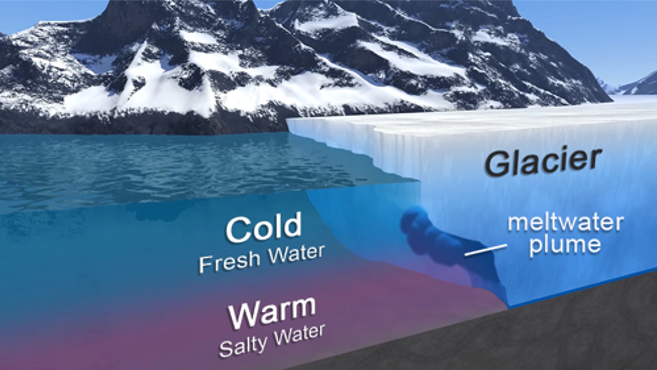
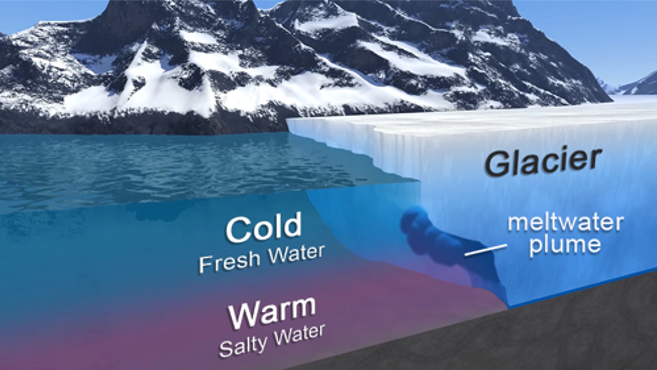
OMG surveys showed that large-scale patterns of ocean temperature variability can persist on the shelf for years. Figure 1 shows locations of all the temperature and salinity profiles collected during a typical yearly ocean survey. The survey was conducted by aircraft and typically took 3-6 weeks to deploy approximately 250 expendable air-launched conductivity, temperature and depth sensors (AXCTDs). Figure 3 shows a subset of these locations along with insets depicting average temperature profiles from three different survey years, over three different regions along the west coast, along with a one-standard error uncertainty bound. The subsurface temperature maximum of approximately 2°C clearly shows the deep, warm layer, and between 2016 and 2018, this layer cooled by almost 1°C in all three regions—a temperature decline that can be traced back through the boundary current, which circulates around the southern half of Greenland (Khazendar et al. 2019). Subsequently, this layer warmed again in recent years in the western region, but not in the northwest.
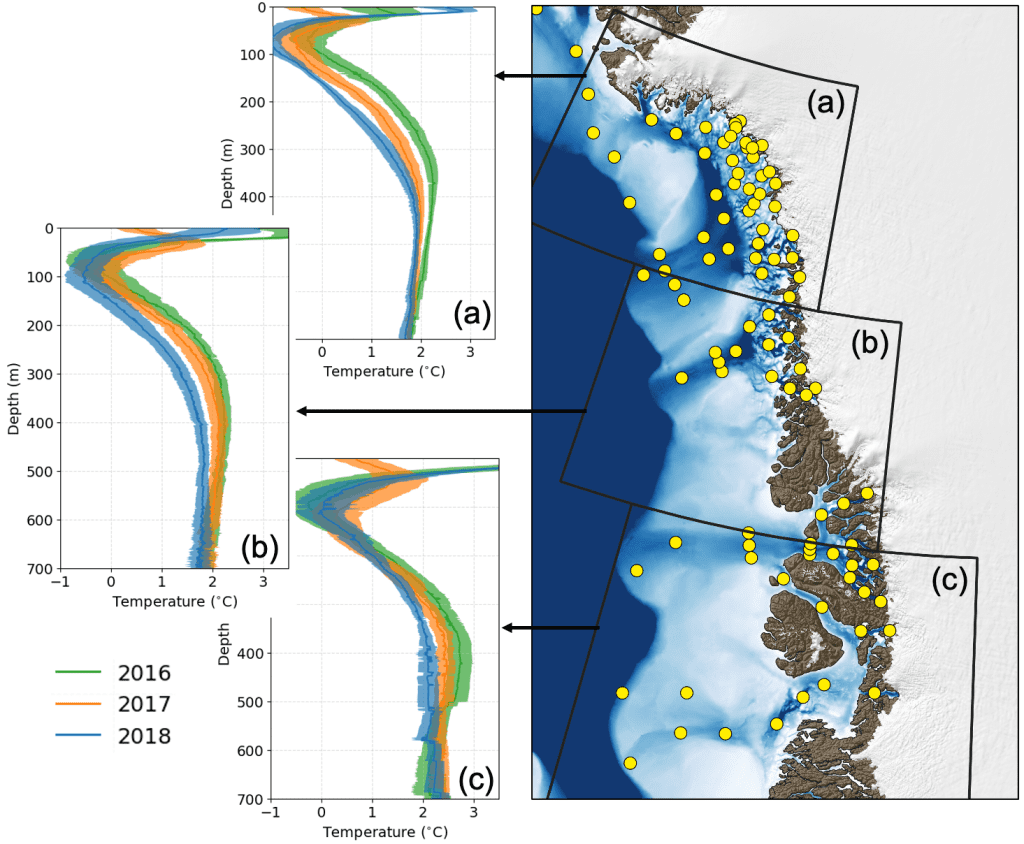
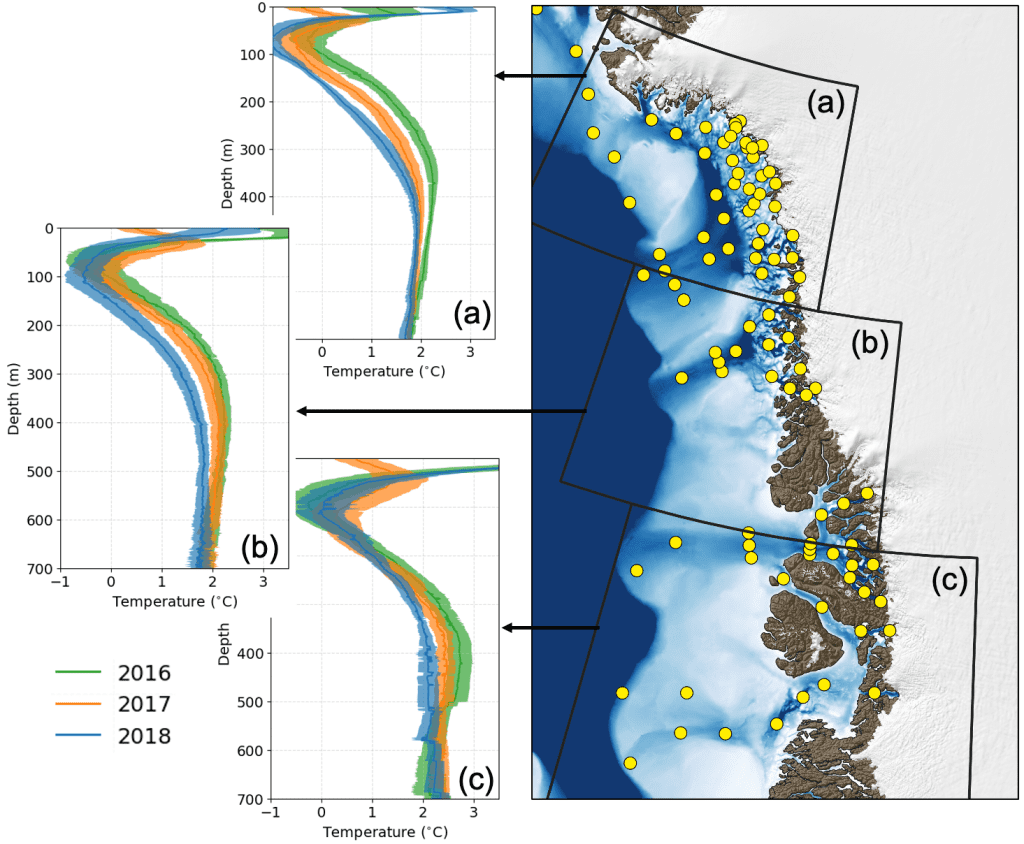
Linking temperature change with ice loss
With more than 70 peer-reviewed publications making use of the observations, the OMG data have revolutionized our knowledge of the coastal bathymetry (Morlighem et al. 2017) and the vulnerability of glaciers to ocean-driven ice loss. In many sectors of the ice sheet, major glaciers with significant potential to raise sea level are susceptible to destabilization by warmer subsurface water. On the central west coast, Greenland’s fastest glacier (Sermeq Kujalleq or Jakobshavn Isbræ) can be dramatically altered by changes in ocean temperature, retreating and accelerating in warming conditions (Holland et al. 2008) and reversing those trends in cooler conditions (Khazendar et al. 2019). Likewise, its northern counterparts Zachariae Isstrøm and Humboldt Gletscher have been retreating and accelerating as a result of increased ocean melt over the past several decades (An et al. 2020; Rignot et al. 2021).
Taken together, OMG’s observations show how ocean conditions surrounding Greenland could change the entire ice sheet’s discharge by as much as a factor of two over the next century and beyond (Wood et al. 2021), which numerical climate projections must account for (Choi et al. 2021). But these measurements also revealed a looming gap: if ocean conditions are driving widespread ice loss in Greenland, how do we continue to observe them after OMG’s end?
OMG floats
During its final years, OMG tested autonomous, Argo-like floats as a tool for monitoring Greenland coastal waters (Fig. 4). Since 2017, seventeen Alamo floats and six APEX floats were deployed on the continental shelf and programmed to collect profiles usually once every 5 days, throughout both summer and winter. Data were collected in roughly the same geographic location by “parking” the floats on the bottom, which limited their drift between profiles.
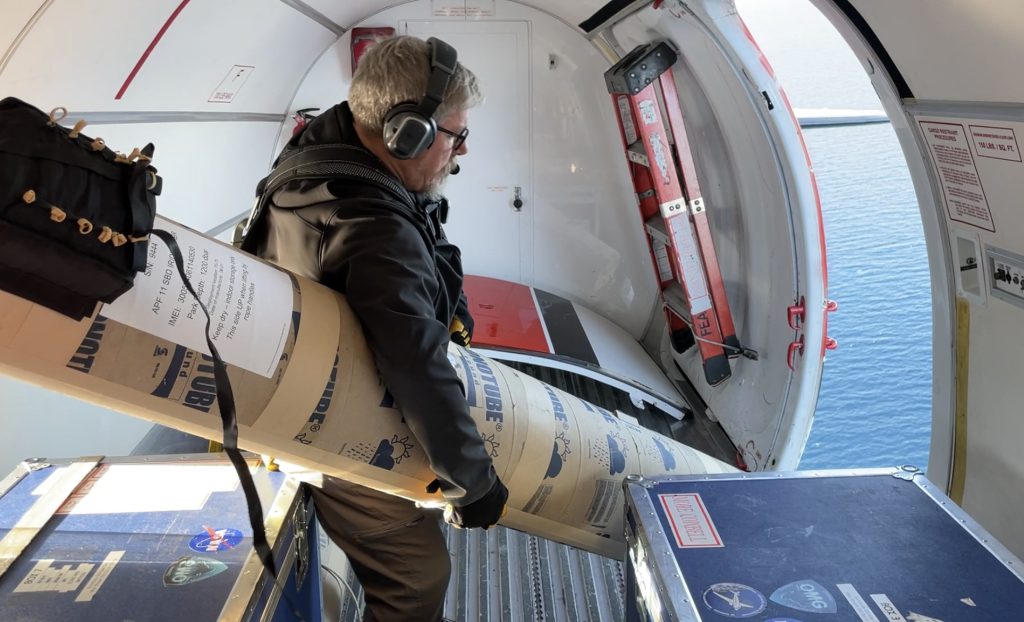
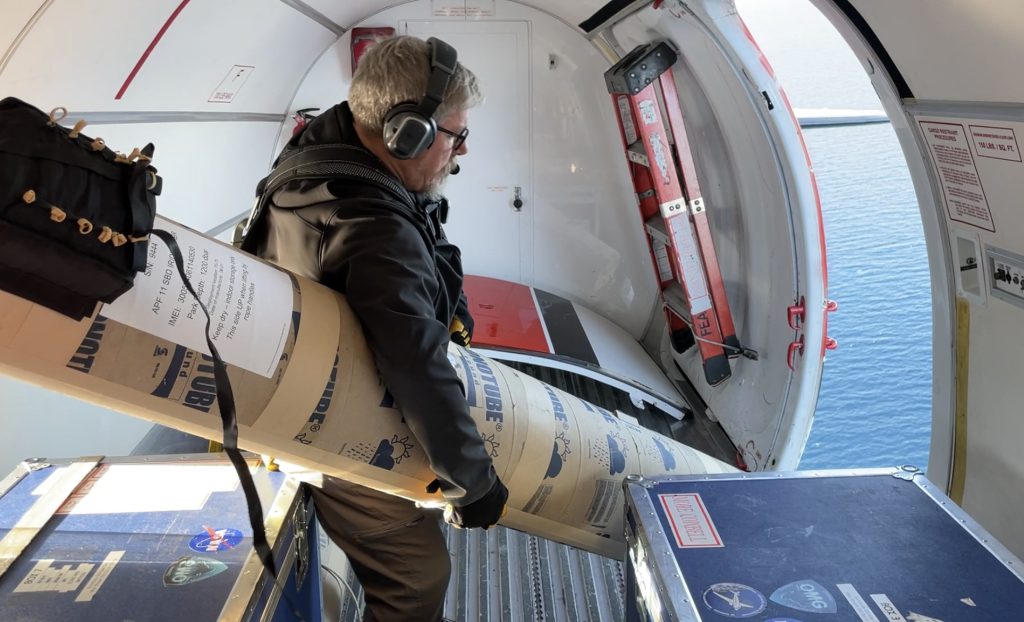
In the first few years, most were lost after a short time due to equipment malfunction or operator error. However, since 2020, four of the APEX floats and two of the Alamo floats not only remained on the shelf but also survived sea ice cover through at least one winter (and in some cases two). In winter, when the floats are unable to surface due to pervasive sea ice, they store data onboard and transmit them later in the summer when access to the surface is again feasible. Although GPS positions are missing for the winter profiles, because the floats park on the shelf, profile positions during the winter can be assigned to those data with high confidence. This small array of floats has begun to build a more robust time series of ocean temperature and salinity in several key regions along Greenland’s west coast (Fig. 5).
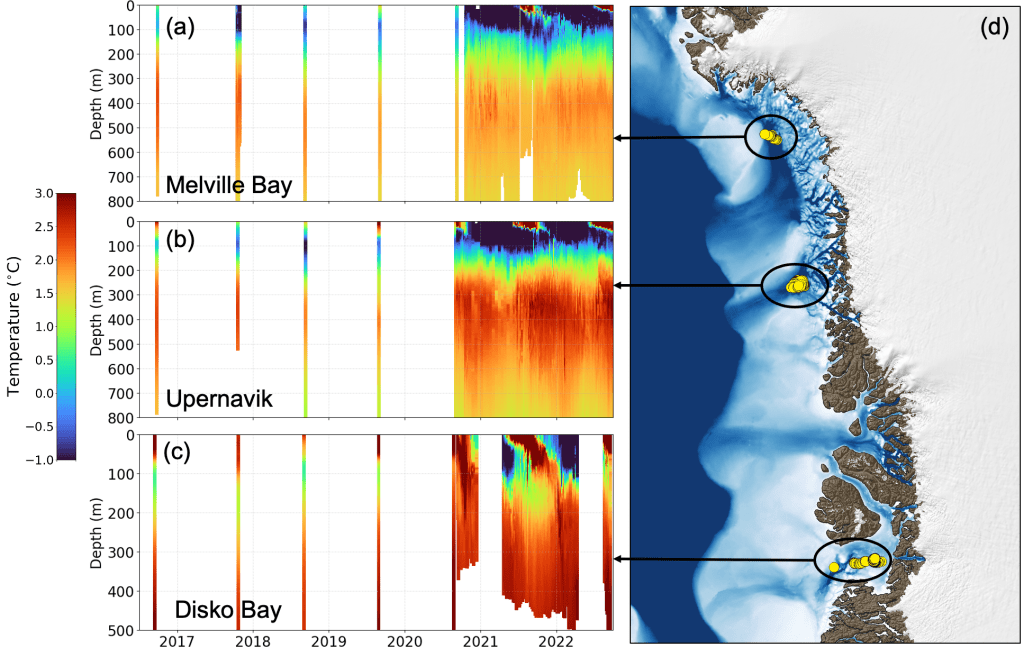
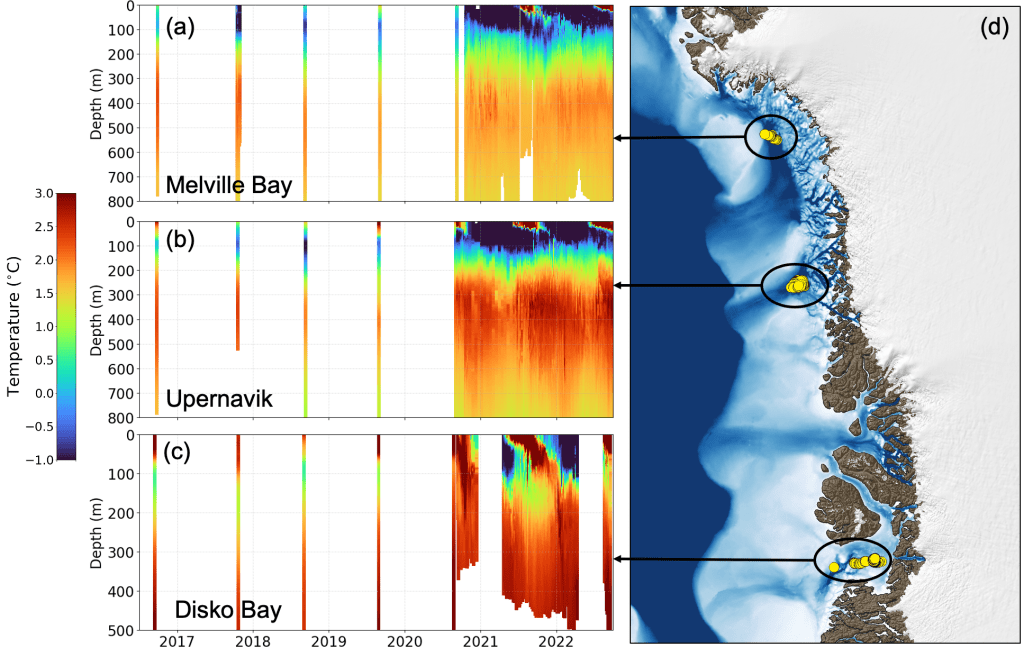
A window to the future
Building on a decade of prior work, OMG demonstrated that the narrow strip of ocean surrounding Greenland provides a window into the future of its ice sheet. Accurate predictions of ice loss and sea level rise will hinge on observation of these waters—especially over interannual to decadal time scales. With the conclusion of OMG, the widespread surveys of the continental shelf have come to an end, and despite their importance, there is no plan to monitor these waters over the long term.
Autonomous floats provide a possible path forward, especially on the west coast where OMG focused its float deployments. Compared to moorings, floats are relatively inexpensive and easier to deploy. Now that OMG has demonstrated their ability to remain on the shelf and survive through the winter, they provide a promising solution for long-term monitoring. Furthermore, the international Argo program has already built infrastructure to maintain an array of more than 4000 of these instruments in the global oceans, and to distribute their data in near real time at no cost. An expansion of the Argo array to include a modest number of floats that park on Greenland’s continental shelf would fill a critical gap in the global climate observing system and would align with recent assessments (Weller et al. 2019; Straneo et al. 2019) that call for sustained ocean observations to serve a variety of scientific and societal purposes.
Methods and data
All data collected during the OMG mission can be found at the Physical Oceanography Distributed Active Archive Center. In Fig. 3, all AXCTD observations from each year’s surveys were averaged together using a simple mean. The band around each mean profile shows the standard error on the mean for each of these estimates. Because each survey was completed in a period of less than 6 weeks, these provide an estimate of the spatial variations of temperature within each region.
Acknowledgments
This work was done at the Jet Propulsion Laboratory, California Institute of Technology under a contract from NASA.
References
An, L., E. Rignot, M. Wood, J. K. Willis, J. Mouginot, and S. A. Khan, 2020: Ocean melting of the Zachariae Isstrøm and Nioghalvfjerdsfjorden glaciers, northeast Greenland. P. Natl. Acad. Sci., 118(2), e2015483118, https://doi.org/10.1073/pnas.2015483118.
Choi, Y., and Coauthors, 2021: Ice dynamics will remain a primary driver of Greenland ice sheet mass loss over the next century. Commun. Earth Env., 2, 26, https://doi.org/10.1038/s43247-021-00092-z.
Holland, D. M., R. H. Thomas, B. De Young, M. H. Ribergaard, and B. Lyberth, 2008: Acceleration of Jakobshavn Isbræ triggered by warm subsurface ocean waters. Nat. Geosci., 1, 659-664, https://doi.org/10.1038/ngeo316.
Khazendar, A., and Coauthors, 2019: Interruption of two decades of Jakobshavn Isbræ acceleration and thinning as regional ocean cools. Nat. Geosci., 12, 277-283, https://doi.org/10.1038/s41561-019-0329-3.
Morlighem, M., and Coauthors, 2017: BedMachine v3: Complete bed topography and ocean bathymetry mapping of Greenland from multibeam echo sounding combined with mass conservation. Geophys. Res. Lett., 44, 11,051- 11,061, https://doi.org/10.1002/2017GL074954.
Rignot, E., and Coauthors, 2021: Retreat of Humboldt Gletscher, north Greenland, driven by undercutting from a warmer ocean. Geophys. Res. Lett., 48, e2020GL091342, https://doi.org/10.1029/2020GL091342.
Snow, T., and Coauthors, 2021: More than skin deep: Sea surface temperature as a means of inferring Atlantic Water variability on the southeast Greenland continental shelf near Helheim Glacier. J. Geophys. Res.-Oceans, 126(4), https://doi.org/10.1029/2020JC016509.
Straneo, F., and P. Heimbach, 2013: North Atlantic warming and the retreat of Greenland’s outlet glaciers. Nature, 504, 36-43, https://doi.org/10.1038/nature12854.
Straneo F., and Coauthors, 2019: The case for a sustained Greenland Ice Sheet-Ocean Observing System (GrIOOS). Front. Mar. Sci., 6, 138, https://doi.org/10.3389/fmars.2019.00138.
Weller R. A., D. J. Baker, M. M. Glackin, S. J. Roberts, R. W. Schmitt, E.S. Twigg, and D. J. Vimont, 2019: The challenge of sustaining ocean observations. Front. Mar. Sci., 6, 105, https://doi.org/10.3389/fmars.2019.00105.
Wood, M., and Coauthors, 2021: Ocean forcing drives glacier retreat in Greenland. Sci. Adv., 7, eaba7282, https://doi.org/10.1126/sciadv.aba7282.
November 23, 2022