M. -L. Timmermans1 and Z. Labe2
1Yale University, New Haven, CT, USA
2Colorado State University, Fort Collins, CO, USA
Highlights
- August mean sea surface temperatures (SSTs) in 2020 were ~1-3°C warmer than the 1982-2010 August mean over most of the Arctic Ocean.
- August mean SSTs show statistically significant warming trends for 1982-2020 in most regions of the Arctic Ocean that are ice-free in August.
- August mean SSTs in 2020 were exceptionally warm in parts of the Kara and Laptev Seas, up to ~5°C warmer than 1982-2010 August mean values, associated with early seasonal ice loss in these regions and anomalously warm surface air temperatures over northern Eurasia.
Introduction
Summer sea surface temperatures (SST) in the Arctic Ocean are driven mainly by the amount of incoming solar radiation absorbed by the sea surface. Solar warming of the Arctic surface ocean is influenced by the distribution of sea ice (with greater warming occurring in ice-free regions), cloud cover, ocean optical properties, and upper-ocean stratification. Discharge of warm Arctic river waters can provide an additional source of heat to the surface marginal seas. In the Barents and Chukchi Seas, there is also a contribution to ocean heat by the advection of warm water from the North Atlantic and North Pacific Oceans, respectively. Arctic SSTs are an essential indicator of the role of the ice-albedo feedback mechanism in any given summer melt season. As the area of sea ice cover decreases (see essay Sea Ice), more incoming solar radiation is absorbed by the ocean and, in turn, the warmer ocean melts more sea ice. In addition, warmer SSTs are associated with delayed autumn freeze-up and increased ocean heat storage throughout the year. Marine ecosystems are influenced by SST, which affects the timing and development of primary and secondary production cycles, as well as available habitat for upper trophic and temperature-sensitive species. Finally, with respect to carbon cycling, warmer SSTs increase the surface-ocean partial pressure of CO2 (pCO2), which reduces the air-ocean pCO2 gradient and therefore the ocean uptake of CO2 from the atmosphere. A study published this year indicates that increased SSTs in recent years may have reduced air-ocean exchange of CO2 by as much as 50% (DeGrandpre et al. 2020).
SST data presented in this section are a blend of in situ and satellite measurements from December 1981 to present, taken from the monthly mean NOAA Optimum Interpolation (OI) SST Version 2 product (OISSTv2) (Reynolds et al. 2002, 2007). Compared to purely in situ temperature measurements, the OISSTv2 product explains about 80% of the variance, with an overall cold bias via its tendency to underestimate SST by 0.02°C (Stroh et al. 2015). The OISSTv2 product uses a linear relationship with sea ice concentration to infer SST, with SST constrained to -1.8°C (the freezing point of seawater with a salinity of 33 g kg-1 at the sea surface) where ice concentration is 100% (Reynolds et al. 2007). Variations in freezing temperature as a result of variations in sea surface salinity (not accounted for in the algorithm) imply that OISSTv2 SSTs under sea ice can be too cool by up to 0.2°C, with the highest errors in the fresher surface waters of the Canada Basin. August mean SSTs provide the most appropriate representation of Arctic Ocean summer SSTs because they are not affected by the cooling and subsequent sea ice growth that typically takes place in the latter half of September. The period 1982-2010 is used as a climatological reference.
August 2020 mean SSTs ranged from 7 to 10°C in the southern Chukchi and Barents Seas to approximately 1 to 3°C in the interior Arctic Ocean marginal seas that are ice-free in August (Fig. 1a). SSTs in August 2020 were consistent with sustained mean August SST warming trends from 1982 to 2020 over much of the Arctic Ocean, with statistically significant (at the 95% confidence interval) linear warming trends of up to +1°C per decade (Fig. 1b); mean August SSTs for the entire Arctic (the Arctic Ocean and marginal seas north of 67° N) exhibit a linear warming trend of 0.03 ± 0.01°C yr-1. The cooling trend in mean August SSTs in the northern Barents Sea region remains a notable exception (see Timmermans et al. 2020).
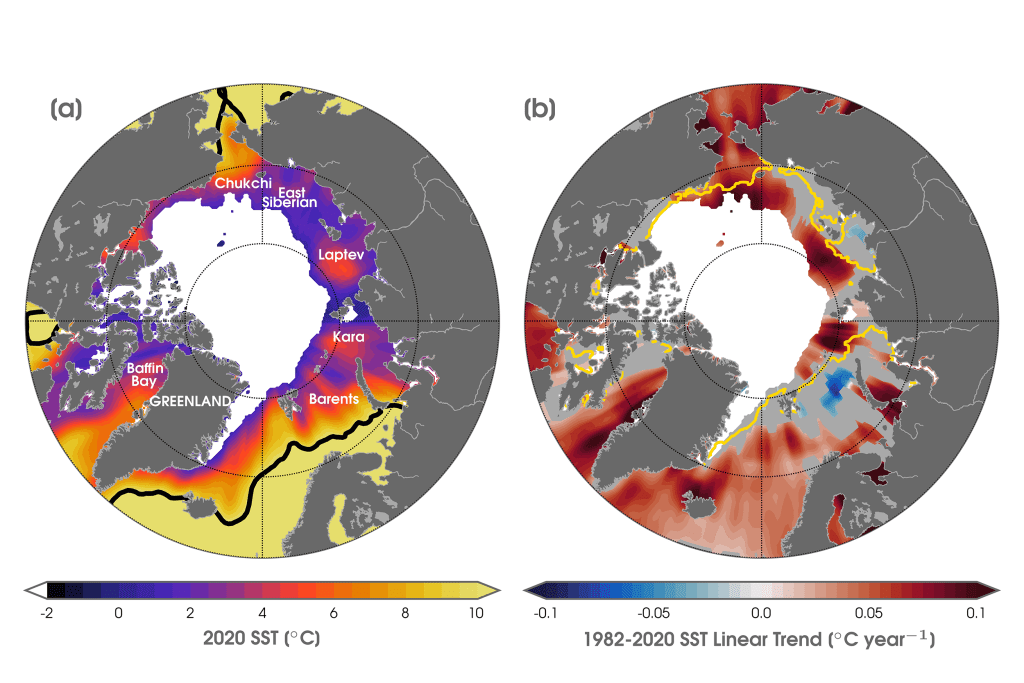
Long-term trends
August 2020 mean SSTs were around 1-3°C warmer than the 1982-2010 August mean over most of the Arctic Ocean’s marginal seas (Fig. 2a). The warmest SSTs were observed in the Laptev and Kara Seas, with values up to 5.5°C warmer than the 1982-2010 August mean (Fig. 2a). Conversely, similar to August 2019 conditions, the northern Barents Sea region was marked by anomalously cool SSTs in August 2020, up to 1.5°C cooler than the 1982-2010 mean (Fig. 2), contributing further to the region’s long-term cooling trend. Relative to August 2019, August 2020 SSTs were up to 4°C cooler in the Chukchi and Beaufort Sea region and a few degrees warmer overall in the Kara and Laptev Seas (Fig. 2b). The strong interannual variability in spatial patterns of SST bear a close relationship to early summer sea ice concentrations, with direct solar heating of the exposed surface waters in regions of low sea ice area, and likely an active ice-albedo feedback.
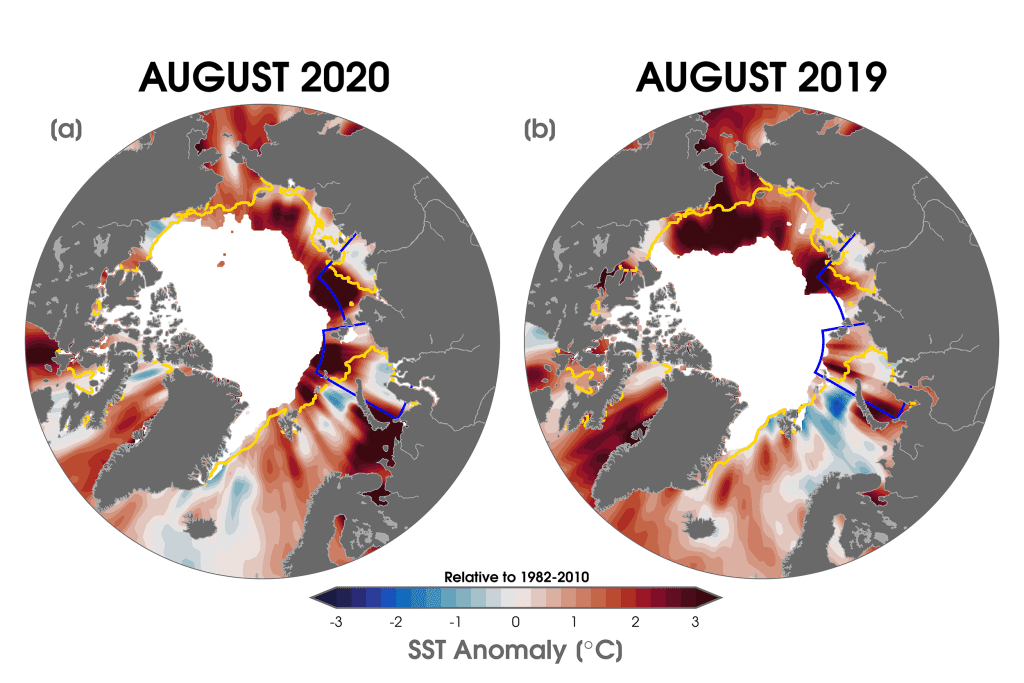
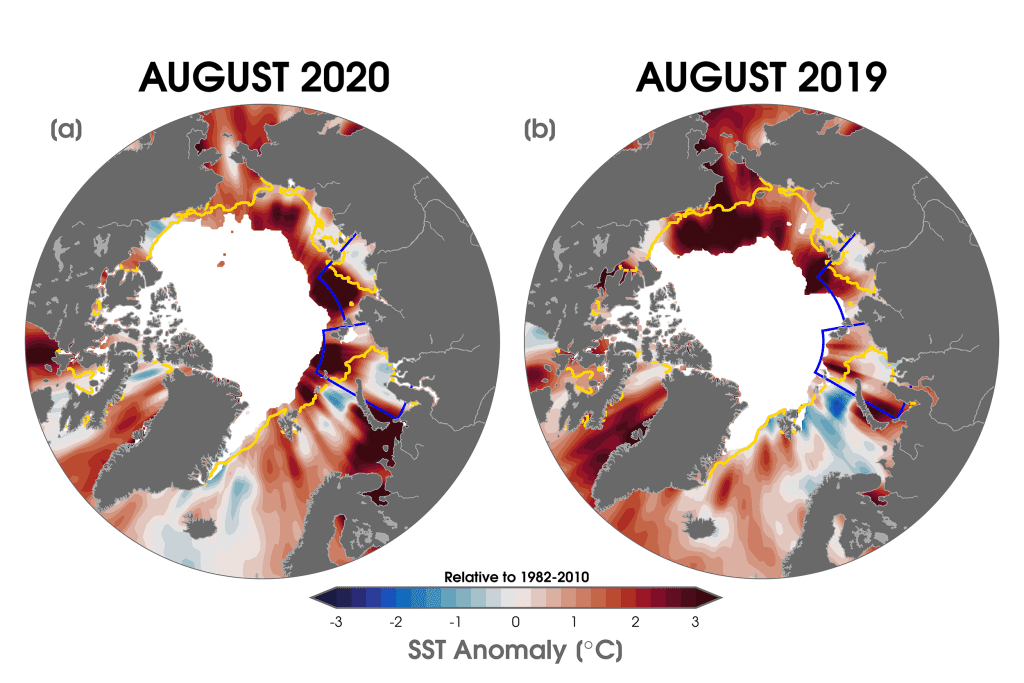
Anomalously warm SSTs in the Laptev and Kara Seas distinguished the August 2020 SST field. Kara Sea SSTs are becoming warmer in August with a linear warming trend over 1982-2020 of 0.03 ± 0.01°C yr-1 (Fig. 3a). Although not statistically significant, Laptev Sea August mean SSTs also appear to be warming, with a linear trend of 0.02 ± 0.02°C yr-1 (Fig. 3b). The interplay between regional sea ice cover and solar absorption is evident in the low sea ice extents in August 2020 in these seas (Fig. 2c,d, and see essay Sea Ice). Both regions also saw exceptionally low sea ice extents in July 2020, with Laptev Sea sea ice extent showing a record minimum for July. In addition, observations indicate increased heat fluxes from the deeper ocean (i.e., warmer waters sourced from the Atlantic Ocean) into the mixed layer in the eastern Eurasian Basin, from around 3-4 W/m2 in 2007-08 to more than 10 W/m2 in 2016-18 (Polyakov et al. 2020). This may also contribute to generally warmer SSTs in the region, although the relative influences of direct solar warming and heat fluxed into the mixed layer from below have not been quantified.
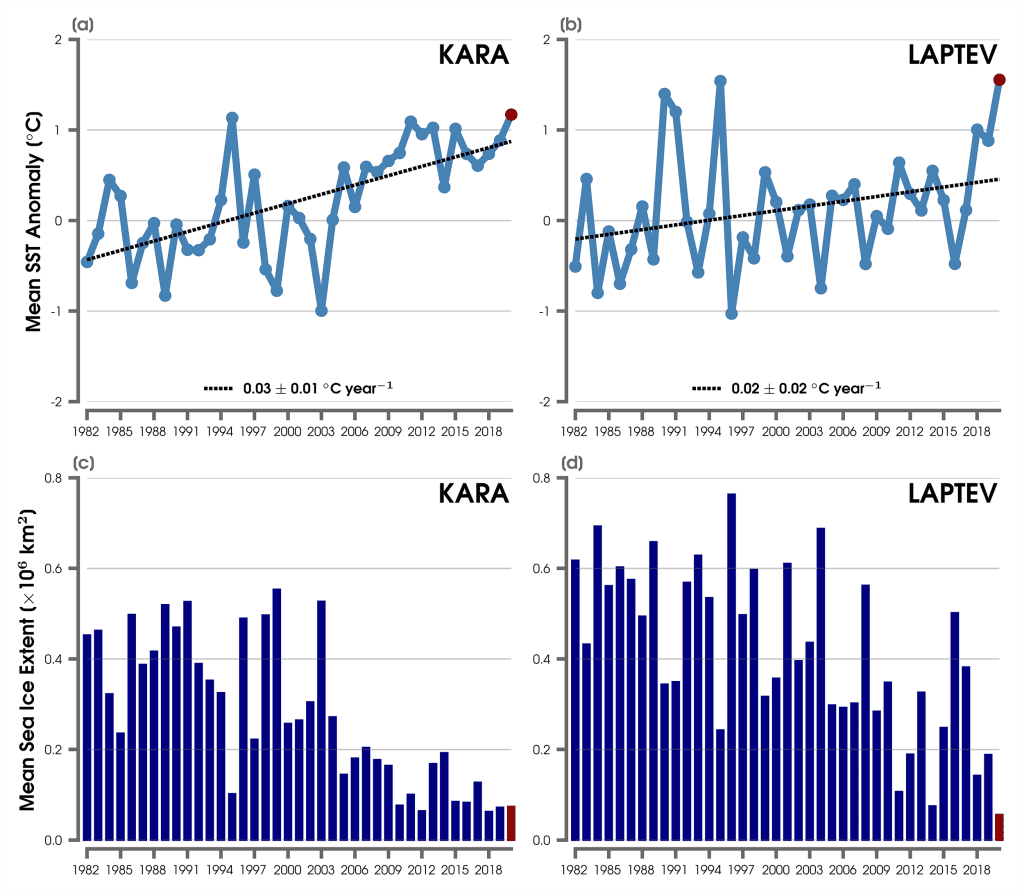
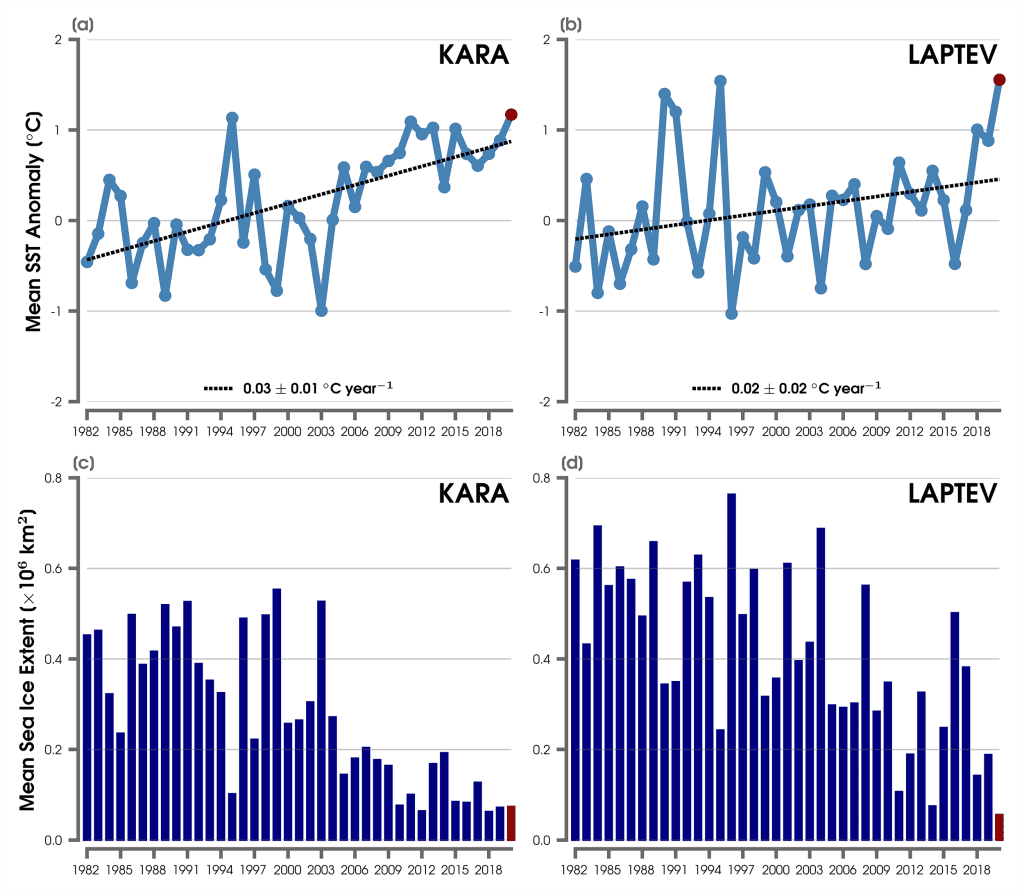
References
DeGrandpre, M., W. Evans, M. -L. Timmermans, R. Krishfield, B. Williams, and M. Steele, 2020: Changes in the arctic ocean carbon cycle with diminishing ice cover. Geophys. Res. Lett., 47, e2020GL088051, https://doi.org/10.1029/2020GL088051.
Fetterer, F., K. Knowles, W. N. Meier, M. Savoie, and A. K. Windnagel, 2017 (updated daily): Sea Ice Index, Version 3: Regional Daily Data. National Snow and Ice Data Center (NSIDC), Boulder, CO, USA, https://doi.org/10.7265/N5K072F8.
Meier, W., J. Stroeve, and F. Fetterer, 2007: Whither Arctic sea ice? A clear signal of decline regionally, seasonally and extending beyond the satellite record. Ann. Glaciol., 46, 428-434, https://doi.org/10.3189/172756407782871170.
Meier, W. N., F. Fetterer, M. Savoie, S. Mallory, R. Duerr, and J. Stroeve. 2017: NOAA/NSIDC Climate Data Record of Passive Microwave Sea Ice Concentration, Version 3. [Indicate subset used]. National Snow and Ice Data Center (NSIDC), Boulder, CO, USA, https://doi.org/10.7265/N59P2ZTG.
Peng, G., W. N. Meier, D. J. Scott, and M. H. Savoie, 2013: A long-term and reproducible passive microwave sea ice concentration data record for climate studies and monitoring. Earth Syst. Sci. Data, 5, 311-318, https://doi.org/10.5194/essd-5-311-2013.
Polyakov, I. V., and Coauthors, 2020: Weakening of the cold halocline layer exposes sea ice to oceanic heat in the eastern Arctic Ocean. J. Climate, 33(18), 8107-8123, https://doi.org/10.1175/JCLI-D-19-0976.1.
Reynolds, R. W., N. A. Rayner, T. M. Smith, D. C. Stokes, and W. Wang, 2002: An improved in situ and satellite SST analysis for climate. J. Climate, 15, 1609-1625, https://doi.org/10.1175/1520-0442(2002)015<1609:AIISAS>2.0.CO;2.
Reynolds, R. W., T. M. Smith, C. Liu, D. B. Chelton, K. S. Casey, and M. G. Schlax, 2007: Daily high-resolution-blended analyses for sea surface temperature. J. Climate, 20, 5473-5496, https://doi.org/10.1175/2007JCLI1824.1, and see http://www.esrl.noaa.gov/psd/data/gridded/data.noaa.oisst.v2.html.
Stroh, J. N., G. Panteleev, S. Kirillov, M. Makhotin, and N. Shakhova, 2015: Sea-surface temperature and salinity product comparison against external in situ data in the Arctic Ocean. J. Geophys. Res. Oceans, 120, 7223-7236, https://doi.org/10.1002/2015JC011005.
Timmermans, M. -L., Z. Labe, and C. Ladd, 2020: Sea surface temperature [in “State of the Climate in 2019”]. Bull. Amer. Meteor. Soc., 101(8), S249-S251, https://doi.org/10.1175/BAMS-D-20-0086.1.
November 9, 2020