L. Mudryk1, A. Elias Chereque2, R. Brown1, C. Derksen1, K. Luojus3, and B. Decharme4
1Climate Research Division, Environment and Climate Change Canada, Ottawa, ON, Canada
2Department of Physics, University of Toronto, Toronto, ON, Canada
3Arctic Research Centre, Finnish Meteorological Institute, Helsinki, Finland
4Centre National de Recherches Météorologiques, Toulouse, France
Highlights
- Extremely high spring 2020 temperatures across Siberia resulted in the lowest June snow extent across the Eurasian Arctic observed in the 54-year satellite record.
- The record Eurasian minimum spring snow extent occurred despite larger than normal winter snowpack accumulation through April.
- Long-term changes in Arctic spring snow extent show similar reductions across both North America and Eurasia. The trend in Arctic peak snow water equivalent differs over North America (negative trend) and Eurasia (little change), but the combined Arctic peak snow water equivalent total is decreasing.
Introduction
Snow covers the Arctic land surface (land areas north of 60° N) for up to 9 months each year, and influences the surface energy budget, ground thermal regime, and freshwater budget of the Arctic (Brown et al. 2017). Snow also interacts with vegetation, affects biogeochemical activity, and influences migration and access to forage for wildlife, which impacts terrestrial and aquatic ecosystems (Callaghan et al. 2011). The assessment provided here is based on an ensemble of datasets derived from satellite observations and reconstructions of snow cover from snowpack models driven by atmospheric reanalyses. Collectively, this basis provides a reliable picture of Arctic snow cover variability over the last five decades.
Snow across the Arctic land surface can be characterized by multiple variables: how much area is covered by snow (snow cover extent – SCE), how long snow remains on the land surface (snow cover duration – SCD), and how much water is stored in solid form by the snowpack (a function of the snow depth and density, commonly expressed as snow water equivalent – SWE). We examine each of these variables in turn for the 2019/20 Arctic snow season.
Snow cover extent
Snow cover extent (SCE) anomalies (relative to the 1981-2010 climatology) for the Arctic in spring 2020 were computed separately for the North American and Eurasian terrestrial sectors of the Arctic. Anomalies were derived from the NOAA snow chart climate data record, which extends from 1967 to present (Fig. 1). SCE anomalies over the Eurasian Arctic were strongly negative in May (4th lowest). Additional melt through June associated with a Siberian heat wave (see essay Surface Air Temperature) resulted in the lowest Eurasian June SCE in the entire 54-year record. North American Arctic spring SCE anomalies in 2020 were below average in both May and June (8th and 10th lowest, respectively).
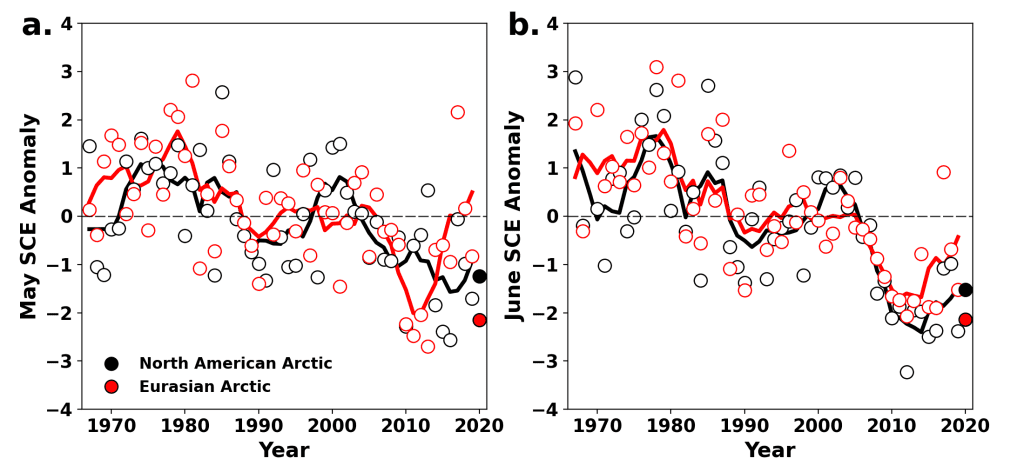
Snow cover duration
Snow cover duration (SCD) anomalies across the Arctic region for the 2019/20 snow season (Fig. 2) were derived from the NOAA daily Interactive Multisensor Snow and Ice Mapping System (IMS) snow cover product, produced at 24 km resolution since 1998. Anomalies in the total number of days with snow cover were computed separately for both halves of the snow season: August 2019 to January 2020, referred to as “onset period,” and February 2020 to July 2020, referred to as “melt period.” SCD during the onset period (Fig. 2a) was close to normal over much of the Arctic, with values reflecting a slightly later start over the eastern Canadian Arctic (Baffin Island and Northern Quebec) and slightly earlier than normal onset over coastal eastern Siberia and the Scandinavian peninsula. The marked difference between Arctic and sub-Arctic Europe is linked to a strong positive mode of the East Atlantic (EA) pattern in atmospheric variability associated with above-average surface temperatures in Europe (see Fig 2a in essay Surface Air Temperature). SCD during the melt period (Fig. 2b) over the North American Arctic indicate a combination of late and early melt with more persistent snow occurring in southern Nunavut and early melt occurring in parts of the northeast (e.g., Baffin Island) and across parts of Alaska and Yukon Territory. Over Eurasia, later than normal melt occurred over the Scandinavian peninsula, likely due to larger than normal accumulation (Fig. 3) from an earlier start to the snow season and above-normal winter precipitation. In marked contrast, and despite the larger than normal accumulation of snow through April (Fig. 3a), springtime temperatures averaging more than 5 degrees above normal (see essay Surface Air Temperature) resulted in snow melting up to a month early across extensive areas of central Siberia.
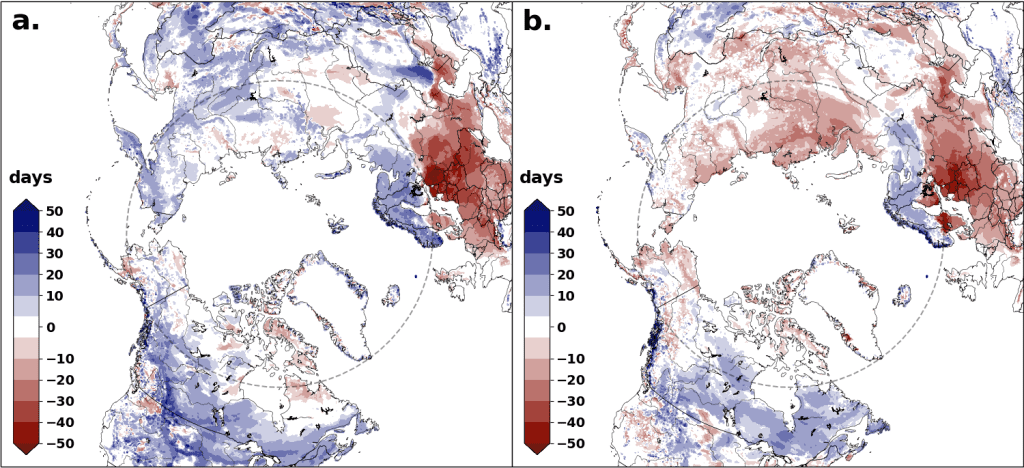
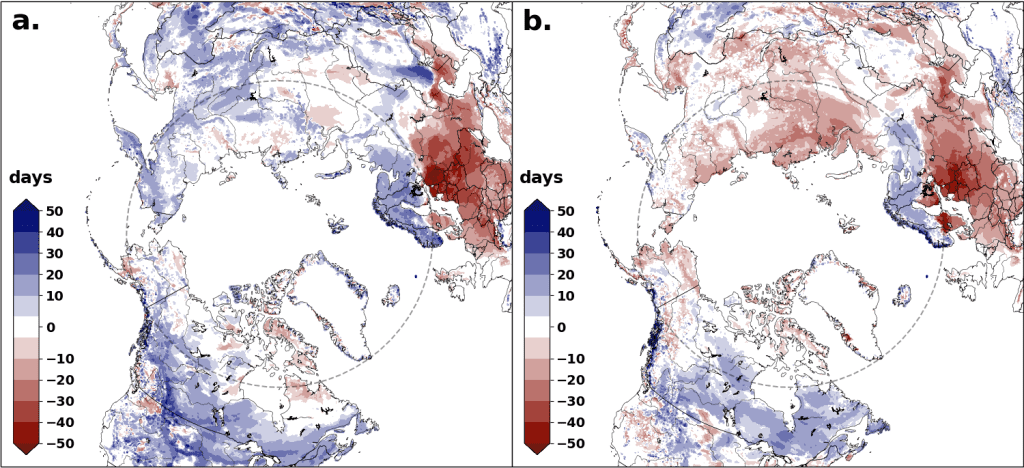
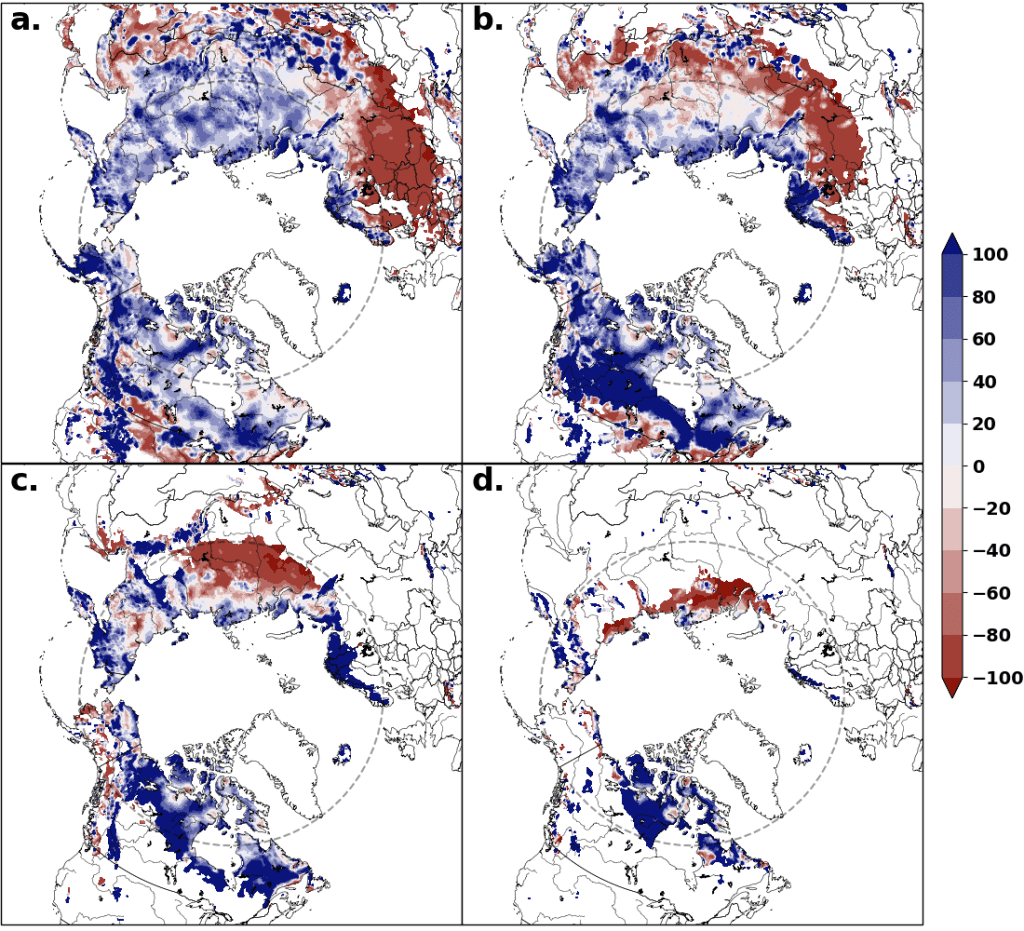
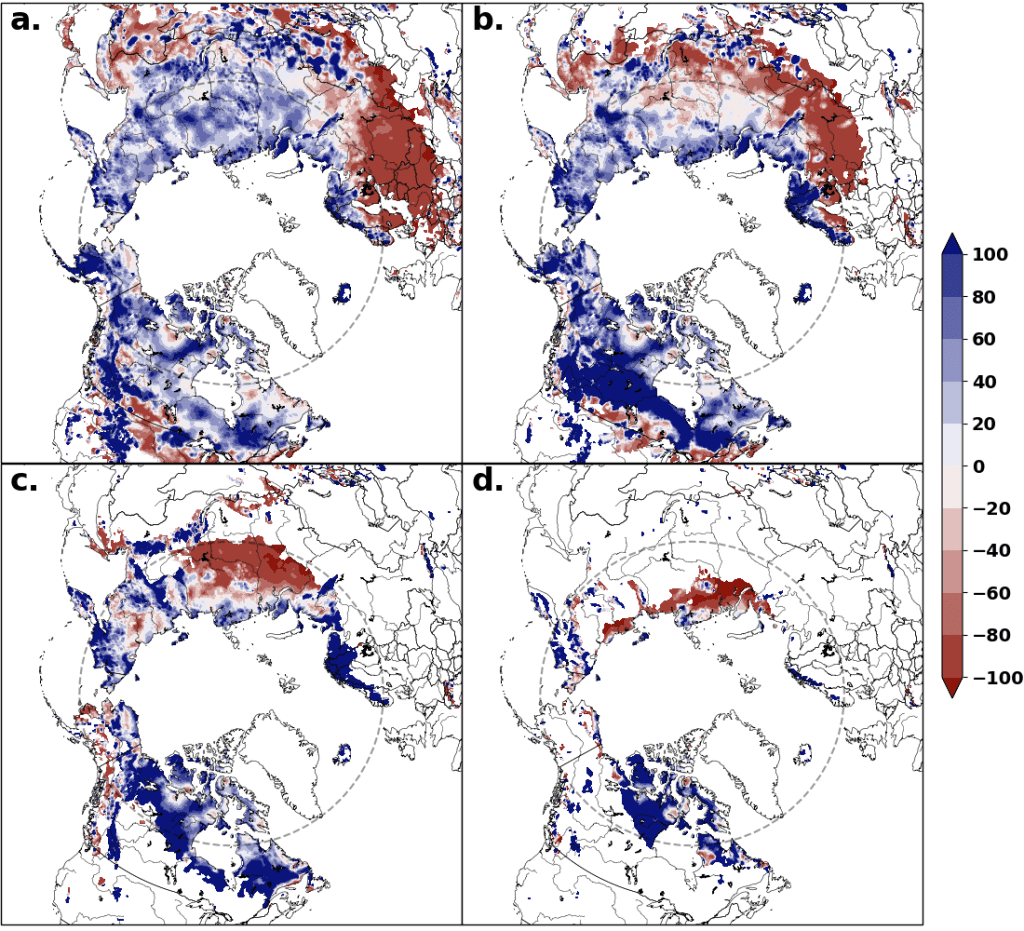
Snow depth
Snow depth anomalies (Fig. 3) were derived from the Canadian Meteorological Centre (CMC) daily gridded global snow depth analysis, which combines air temperature and precipitation analyses with the assimilation of surface snow depth observations. This approach is required to obtain hemispheric estimates of snow depth because in situ observations alone are too spatially sparse to be representative. Snow accumulation over the 2019/20 season resulted in above-average March snow depth across the Arctic. In parts of the North American Arctic, deep snow persisted throughout the spring (Apr-Jun). Across central Siberia, changes in monthly snow depth from April to June signified strong melt, which led to the lowest Eurasian Arctic June snow extent in the full observational record, despite the anomalously deep March snowpack.
Snow water equivalent
Four products were utilized to generate a multi-dataset SWE anomaly time series (1981-2020) for April (Fig. 4): (1) The Modern-Era Retrospective Analysis for Research and Applications version 2 (MERRA-2); (2) a simple temperature index model driven by ERA-interim meteorology described in Brown et al. (2003); (3) the physical snowpack model, Crocus, also driven by ERA-Interim meteorology (Note: Crocus data were not included for 2019 or 2020 due to data availability); and (4) the European Space Agency GlobSnow product derived through a combination of satellite passive microwave data and climate station observations. The use of multiple SWE products allows for the determination of inter-product spread through the time series. SWE estimates for April 2020 indicate above-normal snow accumulation over both the Eurasian and North American Arctic, consistent with deeper-than-normal April snow depths shown in Fig. 3b.
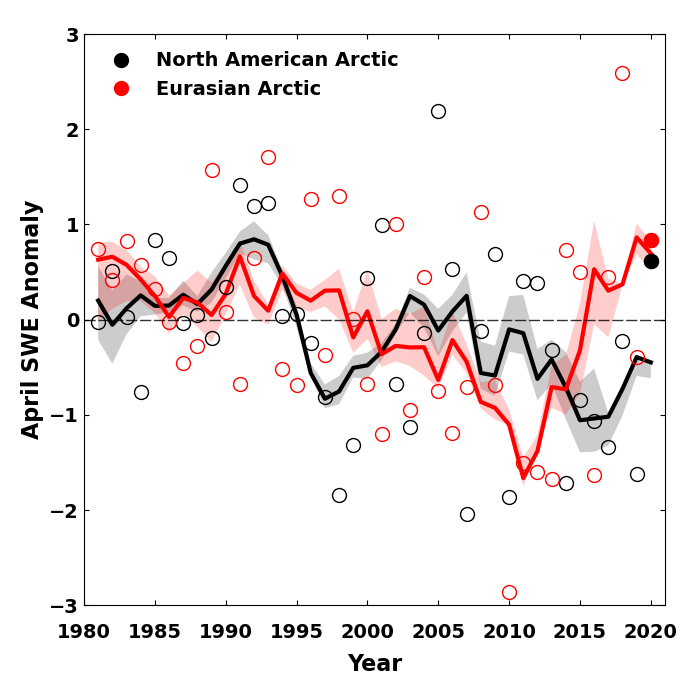
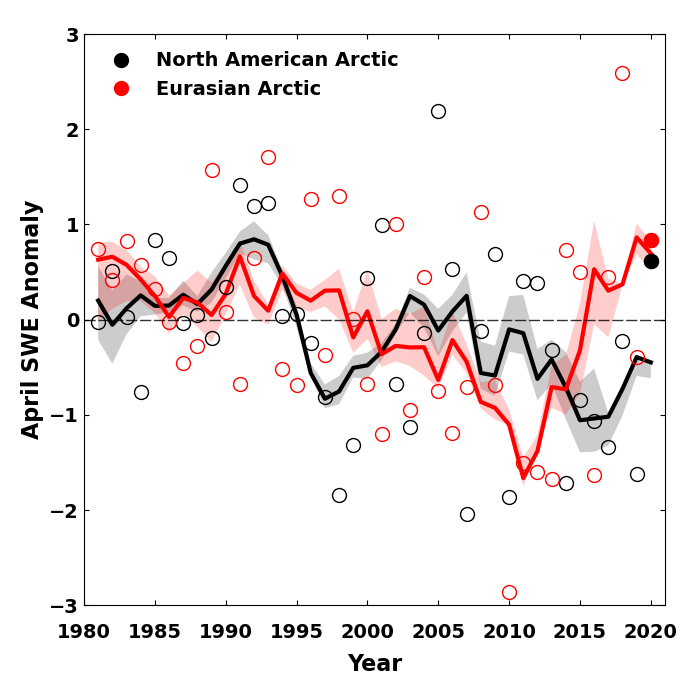
Summary and long-term trends
In summary, snow accumulation during the 2019/20 winter was above normal across the entire Arctic. Nonetheless, extreme springtime temperatures over central Siberia were high enough to result in the lowest Eurasian June SCE documented across the 54-year satellite record. Anomalies of SCE over North America were more moderate, though still negative, due to a combination of regions with deeper, more persistent snow and regions that experienced early melt.
Long-term trends for total Arctic SCE, derived from the data presented in Fig. 1, are negative: -3.7 ± 2.0% decade-1, and -15.5 ± 6.1% decade-1 for May and June, respectively (1981-2020). These trends are on the higher end of estimates from a range of sources, as discussed in Mudryk et al. (2017, 2020). The April trend in Arctic SWE over the 1981-2020 period, calculated from the data presented separately for each continent in Fig. 4, is -2.6 ± 1.8% decade-1, representing a decrease of more than 10% over the entire Arctic since 1981.
References
Brasnett, B., 1999: A global analysis of snow depth for numerical weather prediction. J. Appl. Meteor., 38, 726-740.
Brown, R., B. Brasnett, and D. Robinson, 2003: Gridded North American monthly snow depth and snow water equivalent for GCM evaluation. Atmos.-Ocean., 41, 1-14.
Brown, R. D., and B. Brasnett, 2010: Canadian Meteorological Centre (CMC) Daily Snow Depth Analysis Data, Version 1. Boulder, Colorado USA. NASA National Snow and Ice Data Center Distributed Active Archive Center. https://doi.org/10.5067/W9FOYWH0EQZ3, (last access: 27 July 2020).
Brown, R., D. Vikhamar Schuler, O. Bulygina, C. Derksen, K. Luojus, L. Mudryk, L. Wang, and D. Yang, 2017: Arctic terrestrial snow cover. Snow, Water, Ice and Permafrost in the Arctic (SWIPA) 2017, Arctic Monitoring and Assessment Programme (AMAP), Oslo, Norway, 25-64.
Brun, E., V. Vionnet, A. Boone, B. Decharme, Y. Peings, R. Valette, F. Karbou, and S. Morin, 2013: Simulation of Northern Eurasian local snow depth, mass, and density using a detailed snowpack model and meteorological reanalyses. J. Hydrometeor., 14, 203-219, https://doi.org/10.1175/JHM-D-12-012.1.
Callaghan, T., and Coauthors, 2011: The changing face of Arctic snow cover: A synthesis of observed and projected changes. Ambio, 40, 17-31, https://doi.org/10.1007/s13280-011-0212-y.
Estilow, T. W., A. H. Young, and D. A. Robinson, 2015: A long-term Northern Hemisphere snow cover extent data record for climate studies and monitoring. Earth Sys. Sci. Data, 7(1), 137-142, https://doi.org/10.5194/essd-7-137-2015.
Gelaro, R., and Coauthors, 2017: The Modern-Era Retrospective Analysis for Research and Applications, Version 2 (MERRA-2). J. Climate, 30, 5419-5454, https://doi.org/10.1175/JCLI-D-16-0758.1.
GMAO (Global Modeling and Assimilation Office), 2015: MERRA-2tavg1_2d_lnd_Nx:2d, 1-Hourly, Time-Averaged, Single-Level, Assimilation, Land Surface Diagnostics V5.12.4, Greenbelt, MD, USA, Goddard Earth Sciences Data and Information Services Center (GESDISC), https://doi.org/10.5067/RKPHT8KC1Y1T, (last access: 26 August 2020).
Helfrich, S., D. McNamara, B. Ramsay, T. Baldwin, and T. Kasheta, 2007: Enhancements to, and forthcoming developments in the Interactive Multisensor Snow and Ice Mapping System (IMS). Hydrol. Process., 21, 1576-1586.
Mudryk, L. R., P. J. Kushner, C. Derksen, and C. Thackeray, 2017: Snow cover response to temperature in observational and climate model ensembles. Geophys. Res. Lett., 44, 919-926, https://doi.org/10.1002/2016GL071789.
Mudryk, L., M. Santolaria-Otín, G. Krinner, M. Ménégoz, C. Derksen, C. Brutel-Vuilmet, M. Brady, and R. Essery, 2020: Historical Northern Hemisphere snow cover trends and projected changes in the CMIP6 multi-model ensemble. Cryosphere, 14, 2495-2514, https://doi.org/10.5194/tc-14-2495-2020.
Robinson, D. A., T. W. Estilow, and NOAA CDR Program, 2012: NOAA Climate Data Record (CDR) of Northern Hemisphere (NH) Snow Cover Extent (SCE), Version 1 [r01]. NOAA National Centers for Environmental Information, https://doi.org/10.7289/V5N014G9, (last access: 27 July 2020).
Takala, M., K. Luojus, J. Pulliainen, C. Derksen, J. Lemmetyinen, J. -P. Kärnä, and J. Koskinen, 2011: Estimating Northern Hemisphere snow water equivalent for climate research through assimilation of space-borne radiometer data and ground-based measurements. Remote Sens. Environ., 115, 3517-3529, https://doi.org/10.1016/j.rse.2011.08.014.
U.S. National Ice Center, 2008: IMS Daily Northern Hemisphere Snow and Ice Analysis at 1 km, 4 km, and 24 km Resolutions, Version 1. Boulder, Colorado USA. NSIDC: National Snow and Ice Data Center, https://doi.org/10.7265/N52R3PMC, (last access: 27 July 2020).
November 9, 2020