E. Osborne1, T. Cronin2, J. Farmer3
1NOAA Arctic Research Program, Office of Oceanic and Atmospheric Research, Silver Spring, MD, USA
2U.S. Geological Survey, Reston, VA, USA
3Princeton University – Department of Geosciences, Princeton, NJ, USA
Highlights
- High-resolution Arctic paleo-reconstructions indicate that the magnitude and pace of the 21st century sea-ice decline and surface ocean warming is unprecedented in the last 1,500 years.
- Regions of year-round sea ice likely persisted over much of the past 350,000 years with intermittent ice-free periods until 5,000 years ago, when modern sea ice conditions developed.
- Paleo-reconstructions indicate that Arctic sea ice extent correlates broadly with temperatures and atmospheric CO2 concentrations over geologic time.
The Arctic Ocean is presently experiencing changes in ocean temperature and sea ice extent that are unprecedented in the observational time period (satellite observations: 1979-Present). To provide context for the current changes, scientists turn to paleo records of past climate to document and study natural variability in the Arctic system. Paleoceanographic records that extend limited Arctic instrumental measurements are central to improving our understanding of sea ice dynamics and ocean warming and for enhancing the predictive capability of models. By coupling paleoceanographic records with modern observations, scientists can also contextualize the rate and magnitude of modern change with the deep past.
Proxy Techniques to Investigate Past Ocean Temperature and Sea Ice
Paleoceanographers reconstruct past climate using proxies, or surrogates of past climate variables, preserved in geologic records that have the potential to span millions of years (Table 1). Proxy records are derived from biological, physical or chemical measurements that, for example, can be made using sediment cores (lake or marine), ice cores, or fossil calcium carbonate shells (e.g., corals, foraminifera, ostracodes). Some paleoclimate archives contain relatively direct measurements, such as atmospheric gas concentrations (e.g., CO2, CH4) trapped in bubbles recovered from Antarctic, Greenland and Alpine ice cores. Others can be less direct, such as measuring marine microfossil shell geochemistry to reconstruct past ocean temperature. Proxies are rigorously tested using a number of methods and are often compared to instrumental records to develop an understanding of the proxy.
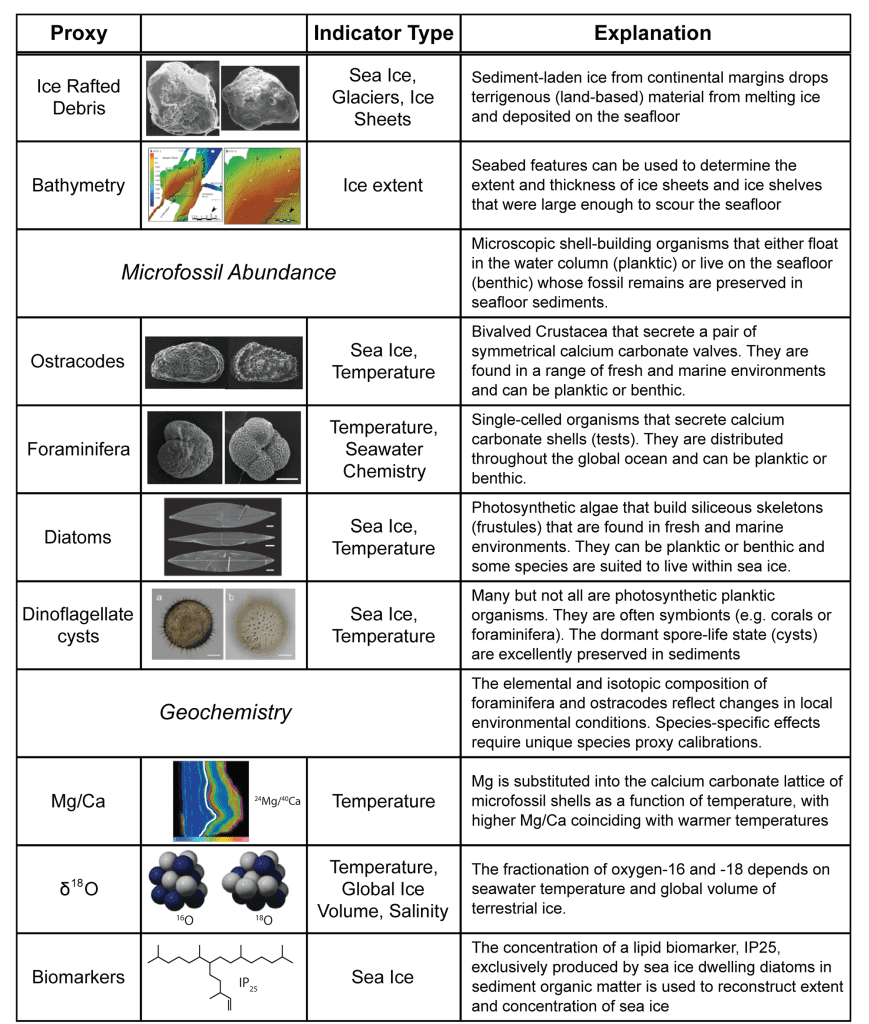
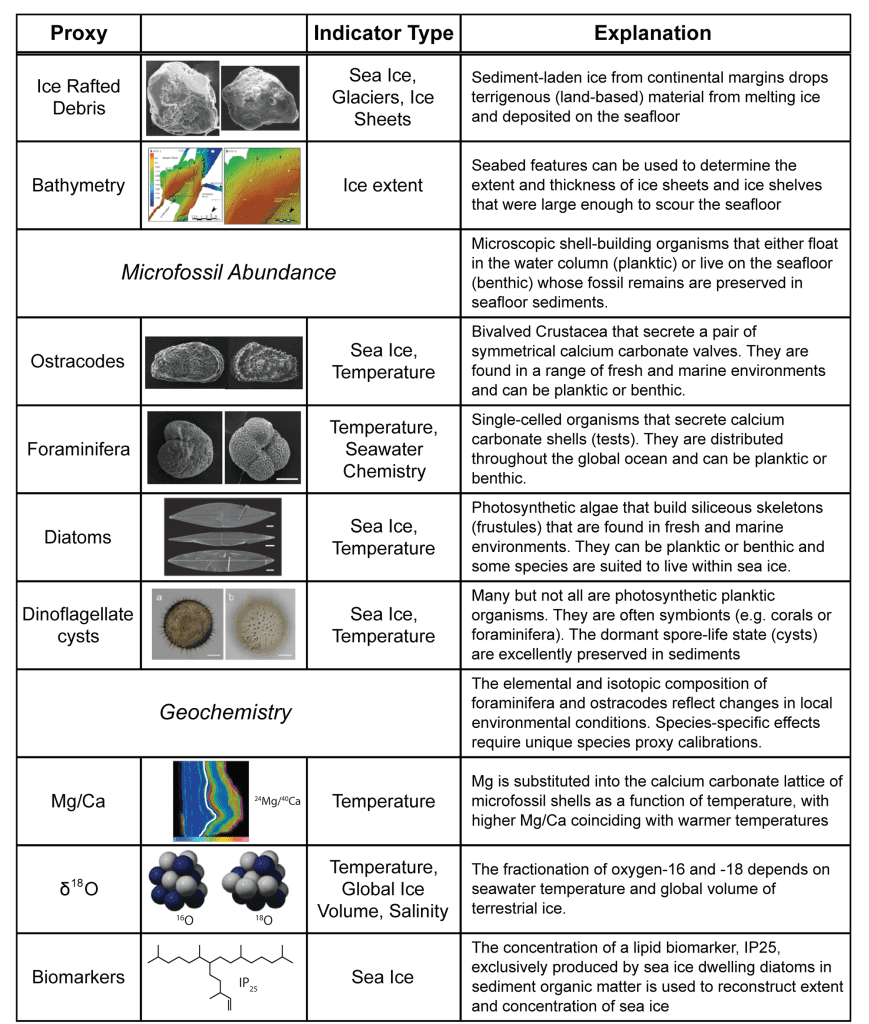
When proxies are applied to core sediment records, they produce reconstructions with variable time resolution based on the study region’s sedimentation rate. For example, sediment cores collected from the central deep-Arctic basin, where sediments accumulate at extremely low rates, have the potential to produce very long-term but low time resolution records. Alternatively, marginal Arctic seas have much higher sedimentation rates due to high primary biological productivity and terrigenous (i.e., derived from land erosion) sediment influx, which facilitates the preservation of proxies in sediments with greater time resolution. Paleoceanographers depend on combinations of high- and low-resolution records to distinguish short- and long-term changes and processes. To further increase the confidence of proxy reconstructions, paleoceanographers often develop multi-proxy records; in these records, more than one proxy technique is used to reconstruct climate variables.
Arctic Paleotemperature and Sea Ice of the Geologic Past
Large seasonal variability of sea ice extent, particularly within marginal seas, is a key characteristic of the Arctic Ocean today. Over geological time, paleoceanographic reconstructions indicate the Arctic has experienced massive sea ice fluctuations from nearly completely ice-free to permanent ice-covered conditions. Much of the understanding of deep-time for the Arctic Ocean has been derived from the first and only successful scientific drilling expedition near the North Pole, Arctic Coring Expedition (ACEX; Integrated Ocean Discovery Program Expedition 302; Fig. 1), in the permanently ice-covered central Arctic (Lomonosov Ridge). The appearance of ice-rafted debris (IRD) and sea-ice dependent diatoms (Synedropsis spp.) in the >400 meters of Arctic marine sediments recovered from ACEX indicate the first Arctic sea ice formed approximately 47 million years ago in the central Arctic (St. John, 2008; Stickley et al., 2009). Global atmospheric CO2 concentration and paleotemperature records indicate that the development of Arctic sea ice coincided with a period of global climate cooling during the middle Eocene (Fig. 2). This transition in global climate is referred to as the shift from Early Cenozoic greenhouse (warm/high CO2) to Late Cenozoic icehouse (cool/low CO2) conditions. The development of year-round (i.e., perennial) sea ice at the North Pole, similar to conditions that exist today, is evident in sediment records as early as 14-18 million years ago (Darby, 2008). These geologic records suggest that transitions in sea ice cover occur over many millennia and often vary in concert with the waxing and waning of circum-Arctic ice sheets and long-term fluctuations in temperature and atmospheric CO2 concentrations (Stein et al., 2012; Jakobsson et al., 2014 for a review).
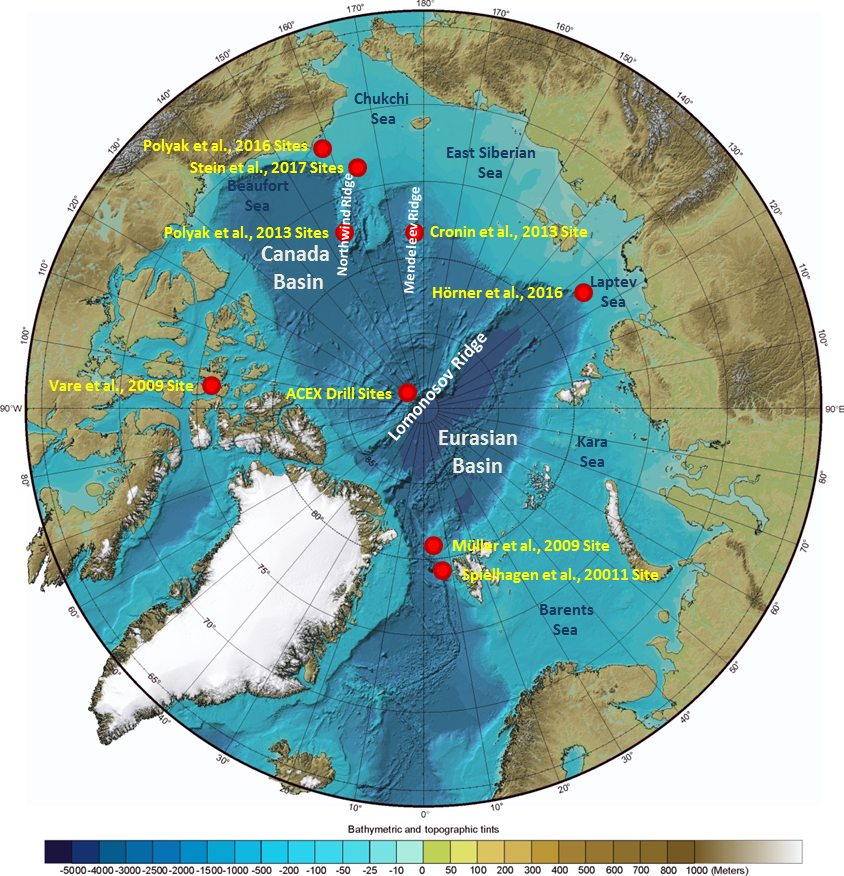
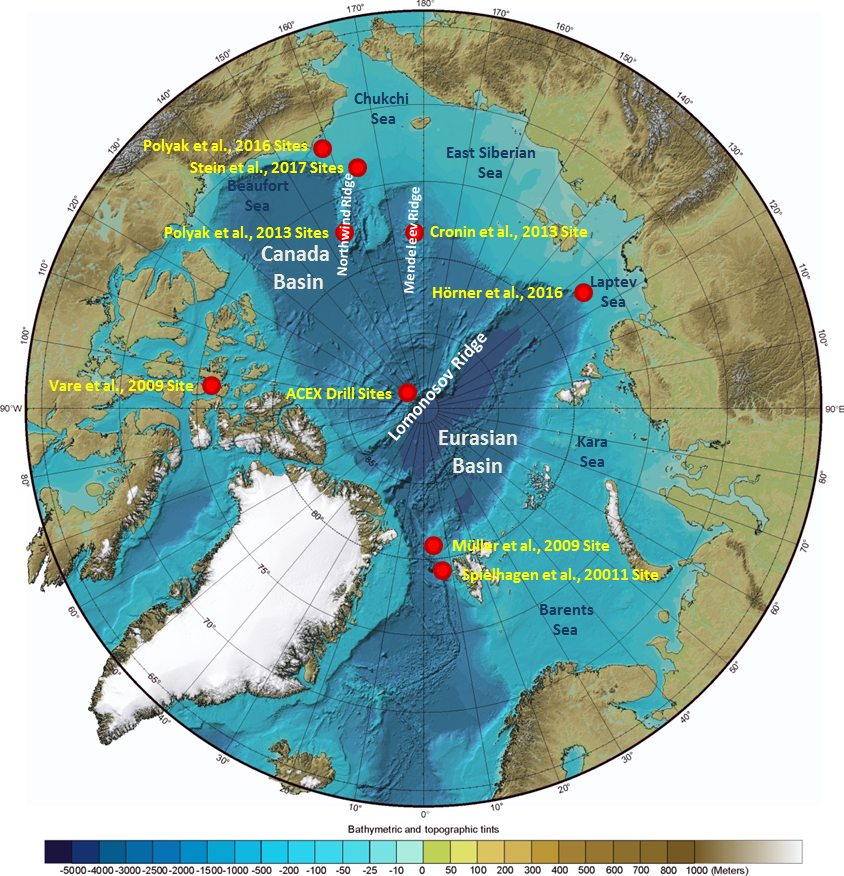
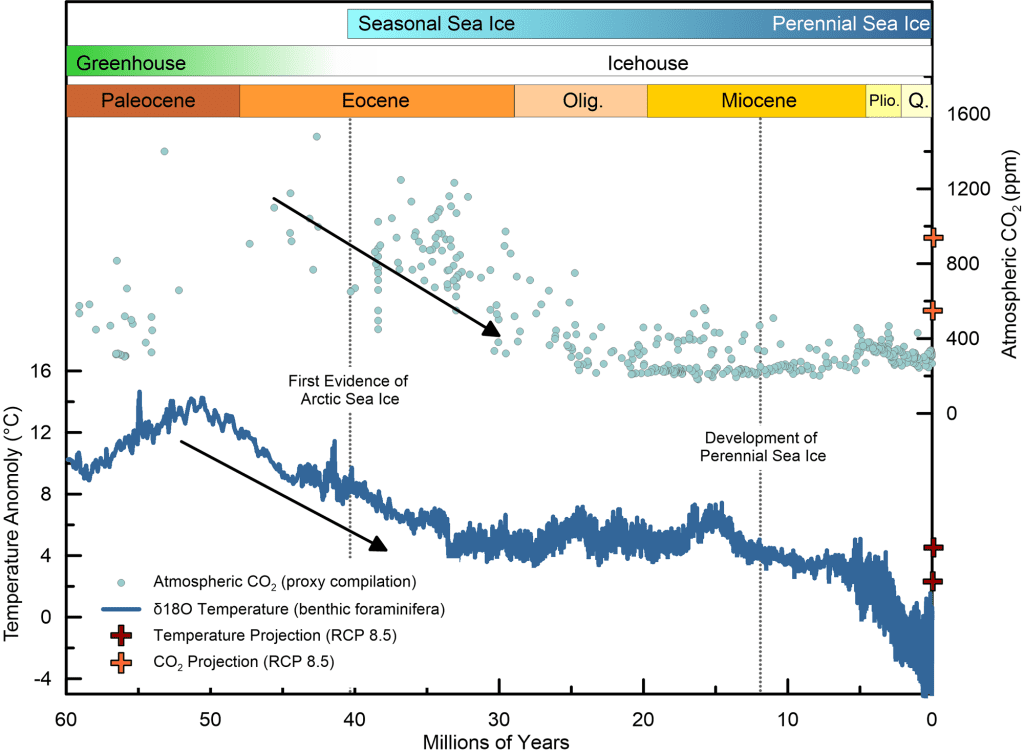
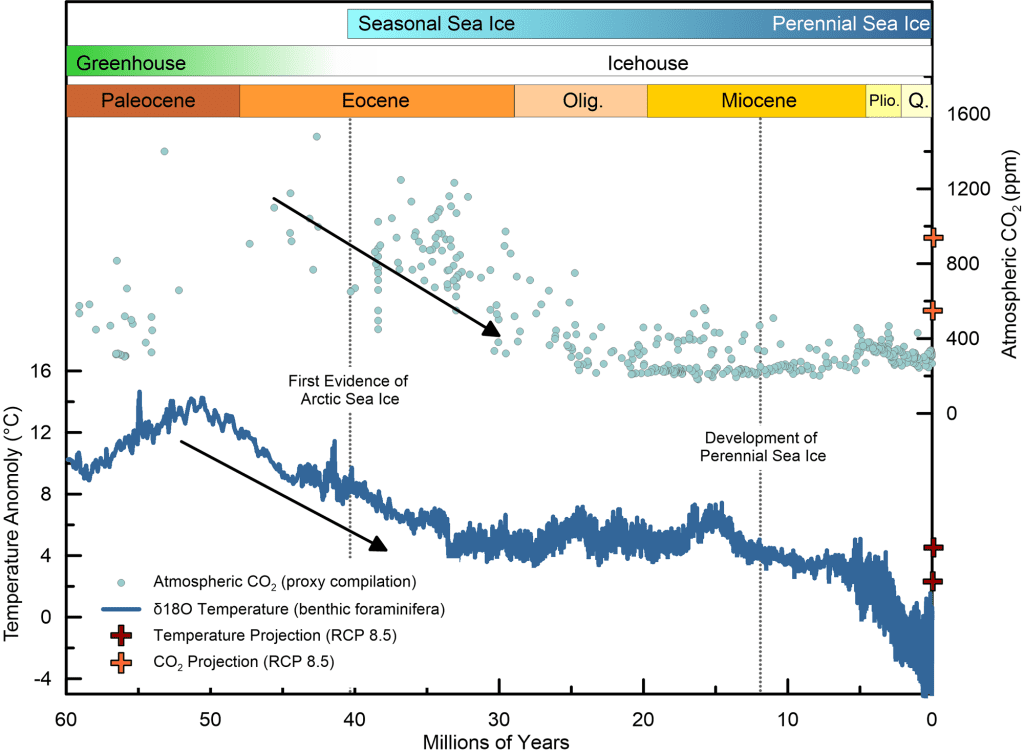
Scientists believe that of the five major oceans, the Arctic experienced the most dramatic environmental change through its Quaternary (2.6 million years ago to present) history. During Quaternary glacial periods, vast glacial ice masses expanded across the entire Arctic Ocean drawing down global sea level, exposing the Arctic’s broad continental shelves and sometimes restricting the exchange between the Arctic and other global oceans. These vast glacial sea-based ice masses, also referred to as ice shelves, developed in concert with the growth of land-based ice sheets. Due to limited preservation of physical evidence, these ice shelves and their connection to circum-Arctic terrestrial ice sheets have been an enigmatic feature of Arctic glacial history. The existence of such ice conditions has been debated since the late 1800s, when a thick floating Arctic ice mass was first proposed. Recently, a geophysical study using multibeam bathymetry allowed researchers to map ice scouring and glacial landforms on the seafloor to constrain the extent and thickness of glacial ice shelves for the first time. This study concluded that a 1-km thick ice shelf covered much of the central Arctic during an extremely strong glacial period 140,000 to 160,000 years ago (Jakobsson et al., 2016). It is estimated that the volume of this particular glacial ice shelf is seven times greater than the total combined volume of all ice shelves on Earth today.
Multi-proxy records of ostracode and foraminifera microfossil assemblages and lithological changes within sediment cores collected on the Northwind and Mendeleev ridges in the western region of the central Arctic were used to reconstruct sea ice variability over the last one million years (Fig. 1). These records indicate that, during this period, perennial sea ice existed as early as 800,000 years ago in the high latitudes of the western Arctic and became a dominant feature 400,000 years ago (Polyak et al., 2013; Cronin et al., 2013). However, it is important to note ice-free conditions have been recorded during this period (e.g., Cronin et al., 2010). These reconstructions also suggest that periods of diminished sea ice in the western Arctic are directly related to enhanced inflow of Pacific water into the central basin (Polyak et al., 2013). In contrast to the western Arctic records, eastern Arctic paleo-records indicate considerably later formation of sea ice (approximately 190,000 years ago; O’Regan et al., 2010). Given that the present-day sea-ice decline is most pronounced in the western Arctic, such regional variability is not surprising, and likely relates to varying inflow of Pacific and Atlantic waters to the Arctic (e.g., Spielhagen et al., 2011; Woodgate et al., 2010).
These records also indicate that over the past tens to hundreds of thousands of years, Arctic sea-ice cover was chiefly governed by ice-age climate cycles (Marzen et al., 2016; Cronin et al., 2010, 2013; Polyak et al., 2013) and also modulated by changes in solar insolation (the amount of solar radiation that reaches the Earth’s surface), ice sheet and ice shelf configuration, atmospheric CO2 concentrations, and inflowing Atlantic and Pacific waters (e.g., Stein et al., 2012).
Arctic Paleotemperature and Sea Ice: The last 10,000 Years
The Holocene interglacial period (11,700 years ago to present) represents the most recent stable warm period, which began after the transitional period of deglaciation (19,000-11,700 years ago). Holocene records of Arctic sea ice extent indicate considerable spatial and temporal variability over the last 10,000 years, including seasonally ice-free periods in various regions from 6,000 to 10,000 years ago when solar insolation in the Arctic was strongest (Polyak et al., 2010; Müller et al., 2012). For many parts of the Arctic, the modern (prior to anthropogenic forcing) perennial sea-ice cover did not fully develop until the latter half of the Holocene, about 5,000 years ago (Darby et al., 2006).
Rapid sediment accumulation in Arctic marginal seas allows for a detailed, higher-resolution history of sea ice variability to be obtained for the Holocene. High-resolution IP25 biomarker (Table 1) records from the Chukchi and East Siberian seas indicate that sea ice concentrations were controlled not only by decreasing insolation, but also by the fraction of Pacific water delivered via the Bering Strait (Stein et al., 2017; Polyak et al., 2016). Records of IP25 from the Fram Strait (Müller et al., 2012), the Laptev Sea (Horner et al., 2016) and the Canadian Arctic Archipelago (Vare et al., 2009) agree with the long-term trends recorded in the Chukchi and Eastern Siberian seas, demonstrating that there was a circum-Arctic Holocene expansion of sea ice in the marginal seas. Lithologic, geochemical and microfossil records from the Fram Strait also indicate regional variations in sea ice related to varying influx of warm Atlantic waters into the Arctic basin (e.g., Werner et al., 2013).
A particularly high-resolution sea ice history has been established for the last 1,450 years using a network of terrestrial (tree ring and lake sediment) and ice-core records located around the margins of the Arctic (Kinnard et al., 2011). This network yields a history of summer sea ice minima during the pre- and post-industrial periods. Results indicate a pronounced decline in summer sea ice beginning in the 20th century with exceptionally low ice extent recorded since the mid-1990s (Fig. 3). While several episodes of reduced and expanded sea ice extent occur in association with climate anomalies such as the Medieval Climate Warm Period (AD 800-1300) and the Little Ice Age (AD 1450-1850), the extent and pace of the modern decline in sea ice is outside of the range of natural variability and unprecedented in the 1450-year reconstruction (Kinnard et al., 2011). The authors conclude that the pre-industrial sea ice climate signal (prior to anthropogenic forcing) was driven by a combination of changes in atmospheric temperature and circulation as well as variations in the inflow of warm saline Atlantic-sourced water masses through the Fram Strait. The significant post-industrial sea ice decline occurs in concert with significant atmospheric and ocean warming driven by an exponential increase in atmospheric CO2 (Fig. 3). High-resolution temperature proxy records from Arctic regions indicate that the modern rate of increasing surface air temperature has not been observed in at least the last 2000 years (McKay and Kaufman, 2014). Further, the Arctic Ocean has experienced intensified warming due to the increasing inflow of relatively warm Atlantic Waters from the Norwegian Sea into the Arctic basin (Fig. 3; Spielhagen et al., 2011). Using both ecology and geochemistry of foraminifera from a Fram Strait sediment core, scientists report a 2° C increase in inflowing water temperature since 1900.
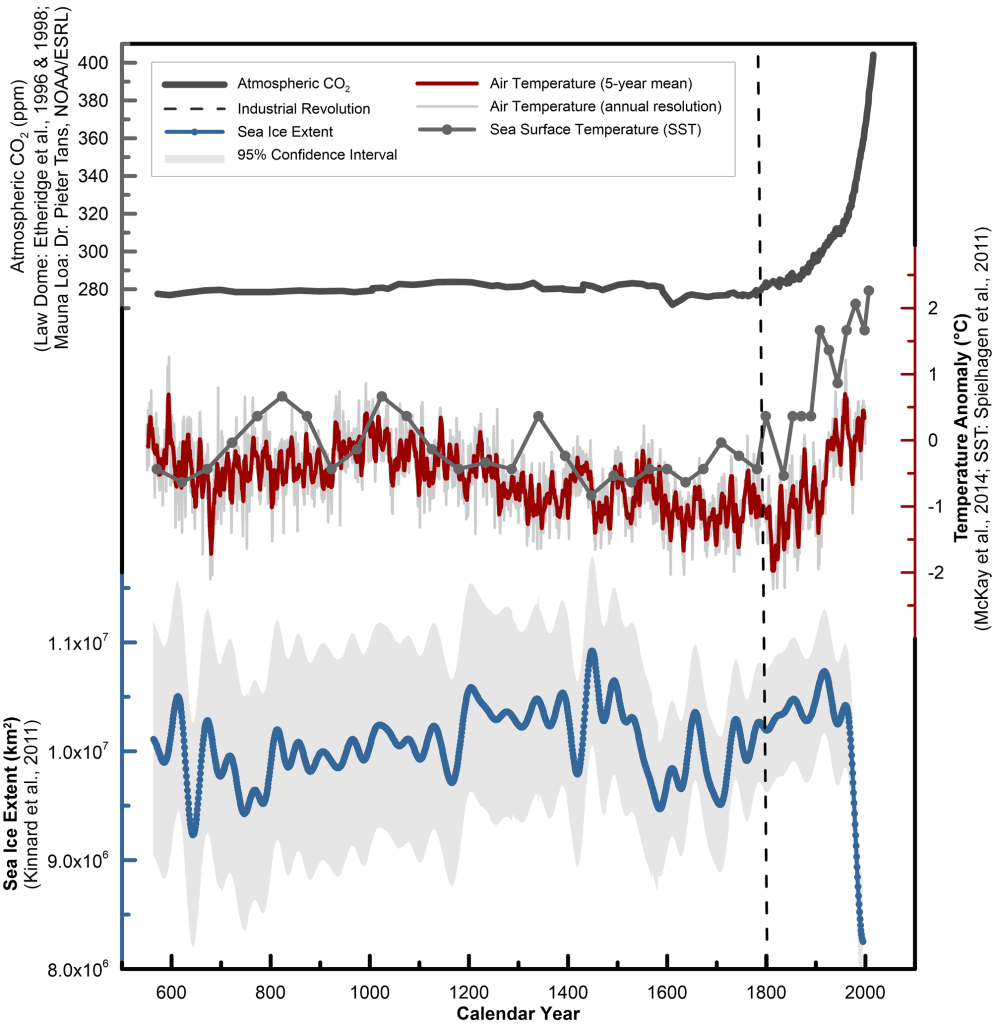
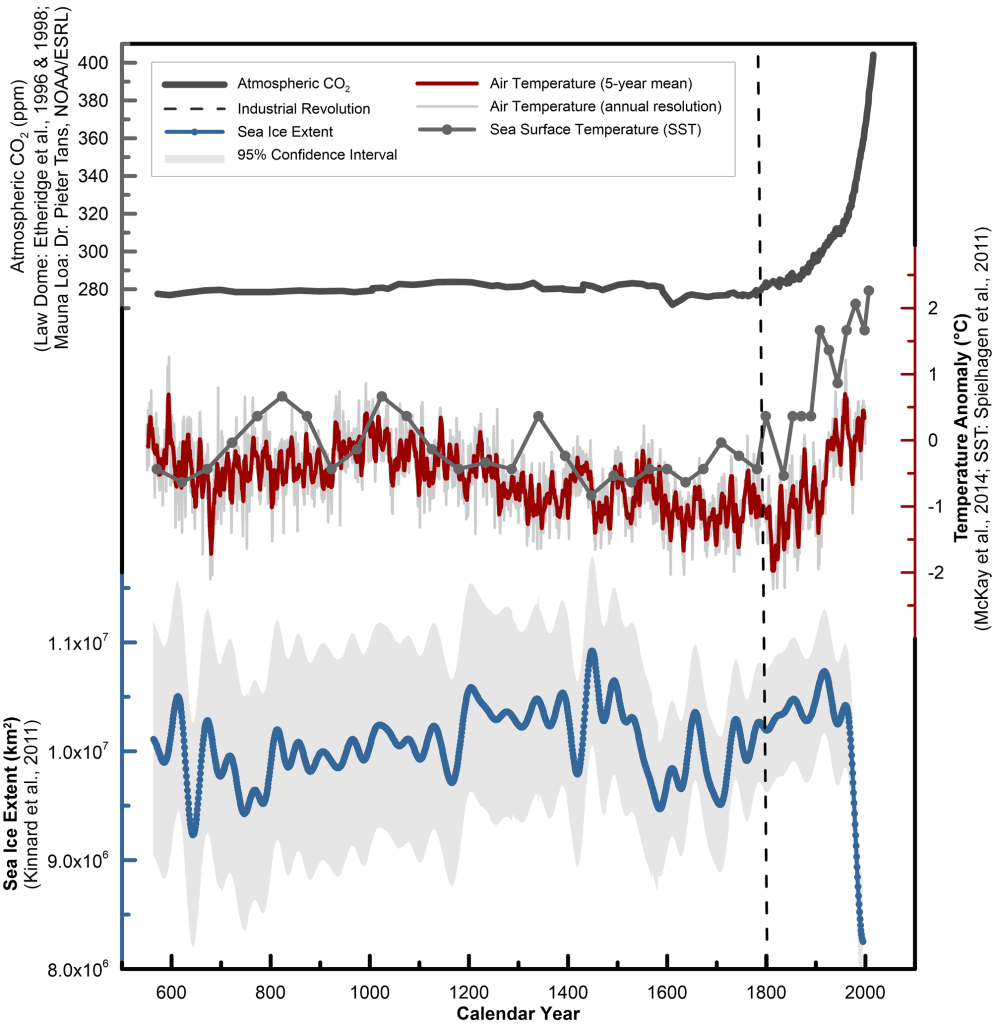
The Arctic plays an important role in the Earth’s climate; therefore, understanding and monitoring Arctic change is important to understanding and predicting global climate impacts. Over time scales of decades to tens of thousands of years, paleoclimate records indicate that sea ice extent over various time scales is forced by insolation, heat advection via ocean water inflows, atmospheric circulation and surface ocean temperatures. Some climate model projections suggest ice-free Arctic summers as early as 2030, and most scientists agree that such conditions are likely before the end of the 21st century. Rapid declines in sea ice extent and thickness reported over the last decades have been attributed to both anthropogenic climate change and natural climate variability. Over the past several decades, inflowing Atlantic waters have warmed and expanded in depth, and river discharge to the Arctic has increased, further driving sea ice melt and warming seas. While understanding the interplay of these factors is critical for future projections of Arctic sea ice and ecosystems, unfortunately most observational time-series records cover only a few decades. This highlights the need for additional paleoceanographic reconstructions of varying spatial and temporal coverage to better understand Arctic Ocean change.
References
Anagnostou, E., E. H. John, K. M. Edgar, et al., 2016: Changing atmospheric CO2 concentration was the primary driver of early Cenozoic climate. Nature, 533, 380-384, doi: 10.1038/nature17423.
Beerling, D. J., and D. L Royer, 2011: Convergent Cenozoic CO2 history. Nature Geoscience, 4, 418-420, doi: 10.1038/ngeo1186.
Cronin, T. M., L. Gemery, W. M. Briggs Jr., M. Jakobsson, L. Polyak, and E. M. Brouwers, 2010: Quaternary sea-ice history in the Arctic Ocean based on a new ostracode sea-ice proxy. Quaternary Science Reviews, 29, 3415-3429.
Cronin, T. M., L. Polyak, D. Reed, E. S. Kandiano, R. E. Marzen, and E. A. Council, 2013: A 600-kyr Arctic sea-ice record from Mendeleev Ridge based on ostracodes. Quaternary Science Reviews, 79, 157-167.
Darby, D. A., L. Polyak, and H.A. Bauch, 2006: Past glacial and interglacial conditions in the Arctic Ocean and marginal seas – a review. Progress in Oceanography, 71, 129-144.
Darby, D. A., 2008: The Arctic perennial ice cover over the last 14 million years. Paleoceanography, doi: 10.1029/2007PA001479.
Etheridge, D. M., L. P. Steele, R. L. Langenfelds, R. J Francey, J. -M. Barnola, and V. I. Morgan, 1996: Natural and anthropogenic changes in atmospheric CO2 over the last 1000 years from air in Antarctic ice and firn. Journal of Geophysical Research, 101, 4115-4128.
Etheridge, D. M., L. P. Steele, R. J. Francey, and R. L. Langenfelds, 1998: Atmospheric methane between 1000 A.D. and present: Evidence of anthropogenic emissions and climatic variability. Journal of Geophysical Research, 103, 15,979-15,993.
Hörner, T., R. Stein, K. Fahl, and D. Birgel, 2016: Post-glacial variability of sea ice cover, river run-off and biological production in the western Laptev Sea (Arctic Ocean): A high-resolution biomarker study. Quaternary Science Reviews, 143, 133-149, doi: 10.1016/j.quascirev.2016.04.011.
Jakobsson, M., K. Andreassen, L. R. Bjarnadottir, et al., 2014: Arctic Ocean glacial history. Quaternary Science Reviews, 92, 40-67, doi: 10.1016/j.quascirev.2013.07.033.
Jakobsson, M., J. Nilsson, L. Anderson, et al., 2016: Evidence for an ice shelf covering the central Arctic Ocean during the penultimate glaciation. Nature Communications, 7, 10365, doi: 10.1038/ncomms10365.
Kinnard, C., C. M. Zdanowicz, D. A. Fisher, E. Isaksson, A. de Vernal, and L. G. Thompson, 2011: Reconstructed change in Arctic sea ice over the last 1,450 years. Nature, 479, 509-512, doi: 10.1038/nature10581.
McKay, N. P., and D. S. Kaufman, 2014: An extended Arctic proxy temperature database for the past 2,000 years. Scientific Data, 1, 140026, doi: 10.1038/sdata.2014.26.
Marzen, R. E., L. H. DeNinno, and T. M. Cronin, 2016: Calcareaous microfossil-based orbital cyclostratigraphy in the Arctic. Quaternary Science Reviews, 149, 109-121.
Müller, J., K. Werner, R. Stein, K. Fahl, M. Moros, and E. Jansen, 2012: Holocene cooling culminates in sea ice oscillations in Fram Strait. Quaternary Science Reviews, 47, 1-14, doi: 10.1016/j.quascirev.2012.04.024.
O’Regan, M., K. St. John, K. Moran, et al., 2010: Plio-Pleistocene trends in ice rafted debris on the Lomonosov Ridge. Quaternary International, 219, 169-176, doi: 10.1016/j.quaint.2009.08.010.
Polyak, L., R. B. Alley, J. T. Andrews, et al., 2010: History of sea ice in the Arctic. Quaternary Science Reviews, 29, 1757-1778.
Polyak, L., K. Best, K. Crawford, E. Council, and G. St-Onge, 2013: Quaternary history of sea ice in the western Arctic Ocean based on foraminifera. Quaternary Science Reviews, 79, 145-156.
Polyak, L., S. T. Belt, P. Cabedo-Sanz, M. Yamamoto, and Y. -H. Park, 2016: Holocene sea-ice conditions and circulation at the Chukchi-Alaskan margin, Arctic Ocean, inferred from biomarker proxies. The Holocene, 26, 1810-1821, doi: 10.1177/0959683616645939.
St. John, K., 2008: Cenozoic ice-rafting history of the central Arctic Ocean: Terrigenous sands on the Lomonosov Ridge. Paleoceanography, 23, PA1S05.
Spielhagen, R. F., K. Werner, S. A. Sorensen, et al., 2011: Enhanced modern heat transfer to the Arctic by warm Atlantic water. Science, 311, 450-453.
Stein, R., K. Fahl, and J. Muller, 2012: Proxy reconstruction of Arctic Ocean sea ice history: ‘From IRD to IP25‘. Polarforschung, 82, 37-71.
Stein, R., K. Fahl, I. Schade, A. Manerung, S. Wassmuth, F. Niessen, S. -I. Nam, 2017: Holocene variability in sea ice cover, primary production, and Pacific-Water inflow and climate change in the Chukchi and East Siberian Seas (Arctic Ocean). Journal of Quaternary Science, 32, 362-379.
Stickley, C. E., K. St. John, N. Koç, R. W. Jordan, S. Passchier, R. B. Pearce, and L. E. Kearns, 2009: Evidence for middle Eocene Arctic sea ice from diatoms and ice-rafted debris. Nature, 460, 376-379.
Vare, L. L., G. Masse, T. R. Gregory, C. W. Smart, and S. T. Belt, 2009: Sea ice variations in the central Canadian Arctic Archipelago during the Holocene. Quaternary Science Reviews, 28, 1354-1366, doi: 10.1016/j.quascirev.2009.01.013.
Werner, K., R. F. Spielhagen, D. Bauch, H. C. Hass, and E. Kandiano, 2013: Atlantic Water advection versus sea-ice advances in the eastern Fram Strait during the last 9 ka: Multiproxy evidence for a two-phase Holocene. Paleoceanography, 28, 283-295, doi: 10.1002/palo.20028.
Woodgate, R. A., T. J. Weingartner, and R. Lindsay, 2010: The 2007 Bering Strait oceanic heat flux and anomalous Arctic sea-ice retreat. Geophysical Research Letters, 37, L01602.
Zhang, Y. G., M. Pagani, Z. Liu, S. M. Bohaty, and R. DeCanto, 2013: A 40-million-year history of atmospheric CO2. Philosophical Transactions of the Royal Society A, 371, 20130096, doi: 10.1098/rsta.2013.0096.
December 4, 2017