H. E. Epstein1, U. S. Bhatt2, M. K. Raynolds3, D. A. Walker3, P. A. Bieniek2, C. J. Tucker4, J. Pinzon4, I. H. Myers-Smith5,
B. C. Forbes6, M. Macias-Fauria7, N. T. Boelman8, S. K. Sweet8
1Department of Environmental Sciences, University of Virginia, Charlottesville, VA, USA
2Geophysical Institute, University of Alaska Fairbanks, Fairbanks, AK, USA
3Institute of Arctic Biology, University of Alaska Fairbanks, Fairbanks, AK, USA
4Biospheric Science Branch, NASA Goddard Space Flight Center, Greenbelt, MD, USA
5School of GeoSciences, University of Edinburgh, Edinburgh, UK
6Arctic Centre, University of Lapland, Rovaniemi, Finland
7School of Geography and the Environment, University Oxford, Oxford, UK
8Lamont-Doherty Earth Observatory, Columbia University, Palisades, NY, USA
Highlights
- Following a general increase over nearly three decades, tundra greenness, derived from remote sensing data, has been declining consistently for the past 2-4 years throughout the Arctic.
- MaxNDVI in 2014 in the Eurasian Arctic and the Arctic as a whole was below the 33-year (1982-2014) average. Temporally-integrated greenness (TI-NDVI) in 2014 had the lowest value on record for Eurasia and the second lowest value for the Arctic as a whole.
- Long-term MaxNDVI and TI-NDVI trends (1992-2014) show tundra “browning” extending over larger areas.
- In contrast to remote sensing observations, field monitoring and experimental studies continue to report increased tundra shrub growth in response to rising air temperatures.
Until recently, the above-ground biomass of Arctic tundra vegetation had been increasing, i.e., vegetation has been “greening”, for at least the past three decades (Bhatt et al. 2013, Frost and Epstein 2014). These vegetation changes have not been spatially homogenous throughout the Arctic (e.g., Bhatt et al. 2013), and they now also appear to be changing direction. In fact, the greenness of above-ground tundra vegetation has been declining for the past 2-4 years (Bhatt et al. 2013; Bieniek et al. 2015). These tundra vegetation changes have implications for numerous aspects of arctic ecosystems, including uptake of atmospheric carbon dioxide through photosynthesis, surface energy and water exchanges, plant-herbivore interactions, the state of the active layer and permafrost, and feedbacks to regional and global climate.
Satellite remote sensing has provided the tool for examining the spatio-temporal patterns of Arctic tundra vegetation change with images dating back to the Cold-War era satellites of the 1960s (Frost and Epstein 2014; Epstein et al. 2015). Providing circumpolar coverage of Arctic vegetation greenness since 1982, the Global Inventory Modeling and Mapping Studies (GIMMS-3g) dataset (GIMMS 2013) is a biweekly, Normalized Difference Vegetation Index (NDVI, an index of photosynthetic activity) time series derived largely from Advanced Very High Resolution Radiometer (AVHRR) sensors aboard NOAA satellites. Two vegetation greenness indices are calculated from the GIMMS-3g: MaxNDVI (maximum annual value) and Time Integrated NDVI (TI-NDVI, sum of the biweekly growing season values of NDVI) (Raynolds et al. 2012; Bhatt et al. 2013). Here we report MaxNDVI and TI-NDVI through 2014, the most recent year for which data are available.
Peak tundra greenness (MaxNDVI) for North America in 2014 ranked 12th and was slightly higher than the mean and mode values for the 33-year record (Fig. 7.1a). However, MaxNDVI for the Arctic as a whole and Eurasia were both below the mean values for the record, ranking 19th and 26th, respectively. MaxNDVI has declined 8.4% since 2011 throughout the Arctic. TI-NDVI for 2014 was extremely low; in fact, it was the lowest year on record for Eurasia, and the second lowest year on record for the Arctic as a whole. TI-NDVI for North America ranked 24th and was slightly below the mean value (Fig. 7.1b). Since 2011, TI-NDVI has decreased 8.7% for the Arctic as a whole and 12% for Eurasia. Other studies corroborate these recent findings. Bhatt et al. (2013) found a significant breakpoint in the TI-NDVI record for Eurasia in 2005, after which the trend became negative. In addition, Piao et al. (2014) found that the strength of the relationship between growing season NDVI and growing season temperature of Arctic ecosystems declined between 1982 and 2011. Finally, from a field perspective, a long-term experimental warming study conducted in Barrow and Atqasuk, Alaska, demonstrated that plant responses to warming surface air temperatures declined between 1994 and 2012 (Kremers et al. 2015).
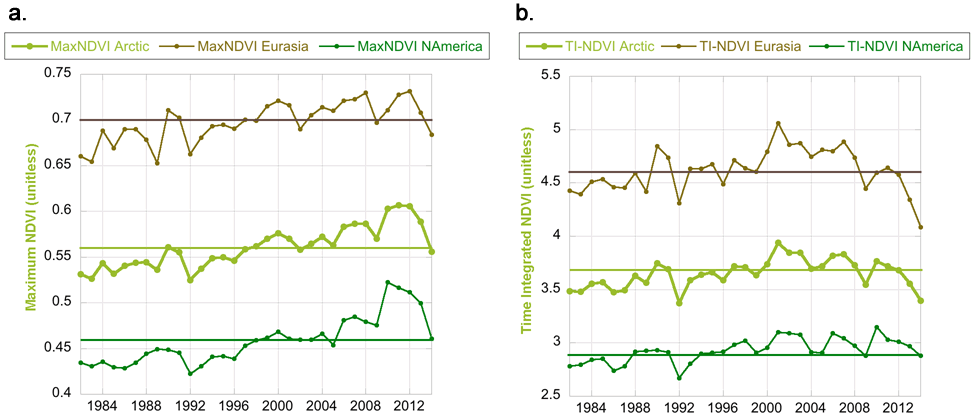
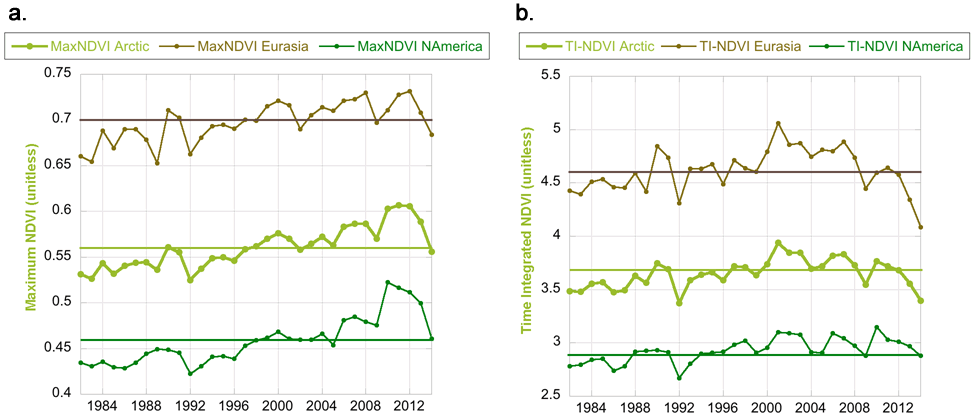
In a more detailed remote sensing analysis, Bieniek et al. (2015) evaluated the long-term trends in bi-weekly NDVI values for the period 1982-2013 in three regions in Alaska, defined according to their adjacent seas (Beaufort, East Chukchi and East Bering). They found that trends in bi-weekly NDVI values were greatest (positive) for the Beaufort Sea region during the peak of the growing season (July and August). For the East Chukchi region, trends in NDVI were positive for most of the growing season (with lower magnitudes than the Beaufort region), with the exception of early in the growing season (May), when trends were negative. For the East Bering region, NDVI trends were only positive late in the growing season (September) and the greatest negative trends were early in the growing season (May and June). The negative NDVI trends during the early part of the growing season were correlated with increasing snow water equivalent values also during May and early June, indicating greater amounts and a longer duration of snow cover. Changes in snow cover (see the essay on Terrestrial Snow Cover) and freeze-thaw events are likely to influence tundra vegetation, particularly shrubs (Bienau et al. 2014; Bjorkman et al. 2015; Boulanger-Lapointe et al. 2014; Hollesen et al. 2015; Preece and Phoenix 2015; Ropars et al. 2015), but the patterns of such climatic changes and the mechanisms by which they affect plants remain unclear.
For the entire period of record (1982-2014), linear trends in MaxNDVI continue to show general circumpolar increases in tundra greenness (Fig. 7.2a). However, since 1992, there are regions of long-term “browning” (decreasing MaxNDVI values) in northwestern Russia, the Yukon Delta region of western Alaska, and the far northern Canadian Arctic Archipelago. Linear trends in TI-NDVI from 1982-2014 also show browning (decreasing TI-NDVI values) in these regions (Fig. 7.2b); however, the vegetation declines extend to Chukotka in northeastern Russia, and to a greater spatial extent in northeastern Siberia, including the Yamal and Taimyr Peninsulas.
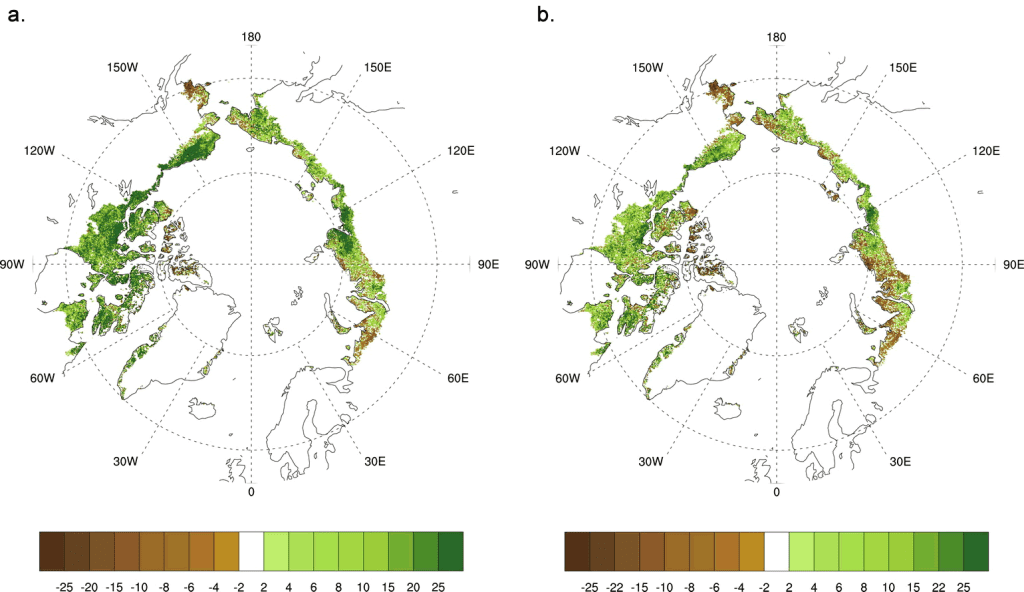
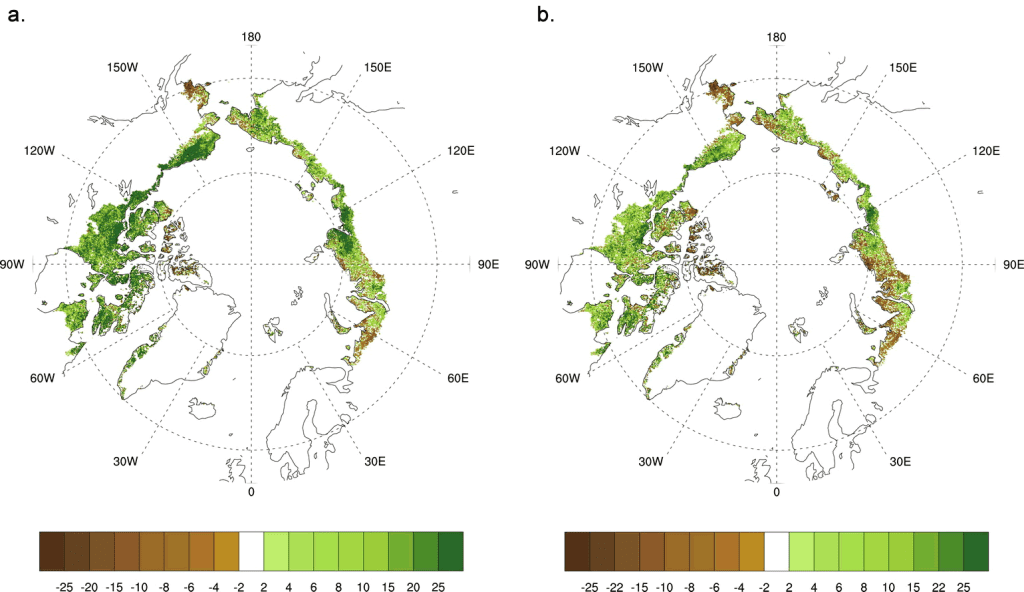
While there are clear indications from remote sensing and some field observations of recent decreases in tundra vegetation greenness, and browning trends occurring over larger areas, a number of field-based studies are still widely reporting increases in the growth of tundra shrubs (with some exceptions, e.g., Bjerke et al. 2014; Ropars et al. 2015). For instance, increased growth of tundra shrubs throughout the Arctic continues to be reported, but, in a new synthesis of tundra shrub dynamics (Myers-Smith et al. 2015), climate variables explained slightly less than 50% of the variation in shrub growth. This synthesis of shrub growth from 37 tundra sites across nine countries, and utilizing ~42,000 annual growth records of 25 species over the past 60 years, demonstrated that the climate sensitivity of shrub growth varied across the tundra biome, with European sites showing greater sensitivity to summer air temperature than North American sites. Sensitivity to air temperature was greater at sites with higher soil moisture content and for taller shrubs (e.g., alders, willows) growing at the edges of their northern or upper elevation ranges. Overall, the climate sensitivity of shrub growth was found to be greatest near the center of the north-south extent of the arctic tundra, where a significant amount of carbon is stored in the permafrost as frozen dead organic matter (Hugelius et al. 2013). Thus, shrub growth is most sensitive to climate in the parts of the tundra biome where the greatest impacts of climate change may occur.
In addition to the Myers-Smith et al. (2015) synthesis, other recently published observational studies (in western Greenland, the western Canadian Arctic and Svalbard) have shown increases in growth by a range of morphological shrub types (prostrate dwarf, erect dwarf and tall) due to both summer warming (van der Wal and Stien 2014; Jørgensen et al. 2015) and winter warming (Fraser et al. 2014; Hollesen et al. 2015), as well as by disturbances caused by wildfires, drained lakes and industrial infrastructure (Fraser et al. 2014). These observational studies show positive responses in both evergreen and deciduous shrubs. Newly published experimental warming studies found greater responses in evergreen shrubs compared to deciduous shrubs; experimentally increased summer air temperatures yielded substantial increases in evergreen shrub cover in Barrow and Atqasuk, Alaska (Hollister et al. 2015) and evergreen shrub above-ground biomass in the central Canadian Low Arctic (Zamin et al. 2014). Based on field data collected from two sites on the North Slope of Alaska (in the vicinity of the Toolik Lake Field Station), Sweet et al. (2015) found that greater deciduous shrub abundance almost tripled the ecosystem net carbon uptake compared to evergreen shrub-graminoid communities due to greater leaf area and a 10-day extension of the period of peak greenness.
References
Bhatt, U. S., D. A. Walker, M. K. Raynolds, P. A. Bieniek, H. E. Epstein, J. C. Comiso, J. E. Pinzon, C. J. Tucker, and I. V. Polyakov, 2013: Recent declines in warming and arctic vegetation greening trends over pan-Arctic tundra, Remote Sensing (Special NDVI3g Issue), 5, 4229-4254; doi:10.3390/rs5094229.
Bienau, M. J., D. Hattermann, M. Kröncke, L. Kretz, A. Otte, W. L. Eiserhardt, A. Milbau, B. J. Graae, W. Durka, and R. Lutz Eckstein, 2014: Snow cover consistently affects growth and reproduction of Empetrum hermaphroditum across latitudinal and local climate gradients. Alpine Botany, 124, 115-129.
Bieniek, P. A., U. S. Bhatt, D. A. Walker, M. K. Raynolds, J. C. Comiso, H. E. Epstein, J. E. Pinzon, C. J. Tucker, R. L. Thoman, H. Tran, N. Mölders, M. Steele, J. Zhang, and W. Ermold, 2015: Climate drivers linked to changing seasonality of Alaska coastal tundra vegetation productivity. Earth Interactions, in press.
Bjerke, J. W., S. R. Karlsen, Høgda, E. Malnes, J. U. Jepsen, S. Lovibond, D. Vikhamar-Schuler, and H. Tømmervik, 2014: Record-low primary productivity and high plant damage in the Nordic Arctic Region in 2012 caused by multiple weather events and pest outbreaks, Environ. Res. Lett., 9, 084006.
Bjorkman, A. D., S. C. Elmendorf, A. L. Beamish, M. Velland, and G. H. R. Henry, 2015: Contrasting effects of warming and increased snowfall on Arctic tundra plant phenology over the past two decades, Global Change Biol., doi:10.1111/gcb.13051.
Boulanger-Lapointe, N., E. Lévesque, S. Boudreau, G.H.R. Henry, and N. Martin Schmidt, 2014: Population structure and dynamics of willow (Salix arctica) in the High Arctic. J. Biogeogr., 41, 1967-1978.
Elmendorf, S. C., G. H. R. Henry, R. D. Hollister, A. M. Fosaa, W. A. Gould, L. Hermanutz, A. Hofgaard, I. S. Jónsdóttir, J. C. Jorgenson, E. Lévesque, B. Magnusson, U. Molau, I. H. Myers-Smith, S. F. Oberbauer, C. Rixen, C. E. Tweedie, and M. D. Walker, 2015: Experiment, monitoring, and gradient methods used to infer climate change effects on plant communities yield consistent patterns. Proc. Nat. Acad. Sci., 112, 448-452.
Epstein, H. E., G. V. Frost, D. A. Walker, and R. Kwok, 2015: The Arctic – Declassified high-resolution visible imagery for observing the Arctic. In, State of the Climate in 2014. Bull. Amer. Meteorol. Soc., 96, S142-S143.
Fraser, R. H., T. C. Lantz, I. Olthof, S. V. Kokelj, and R.A. Sims, 2014: Warming-induced shrubs expansion and lichen decline in the western Canadian Arctic. Ecosystems, 17, 1151-1168.
Frost, G. V. and H. E. Epstein, 2014: Tall shrub and tree expansion in Siberian tundra ecotones since the 1960s. Global Change Biol., 20, 1264-1277.
Global Inventory Modeling and Mapping Studies (GIMMS), 2013: Available online: http://gcmd.nasa.gov/records/GCMD_GLCF_GIMMS.html.
Hollesen, J., A. Buchwal, G. Rachlewicz, B. U. Hansen, M. O. Hansen, O. Stecher, and B. Elberling, 2015: Winter warming as an important co-driver for Betula nana growth in western Greenland during the past century. Global Change Biology, 21, 2410-2423.
Hollister, R. D., J. L. May, K. S. Kremers, C. E. Tweedie, S. F. Oberbauer, J. A. Liebig, T. F. Botting, R. T. Barrett, and J. L. Gregory, 2015: Warming experiments elucidate the drivers of observed directional changes in tundra vegetation. Ecology and Evolution, 5, 1881-1895.
Hugelius, G., J. G. Bockheim, P. Camill, B. Elberling, G. Grosse, J. W. Harden, K. Johnson, T. Jorgenson, C. D. Koven, P. Kuhry, G. Michaelson, U. Mishra, J. Palmtag, C. L. Ping, J. O’Donnell, L. Chirmeister, E. A. G. Schuur, Y. Sheng, L. C. Smith, J. Strauss, and Z. Yu, 2013: A new data set for estimating organic carbon storage to 3m depth in soils of the northern circumpolar permafrost region. Earth System Sci. Data, 5, 393-402.
Jørgensen, R. H., M. Hallinger, S. Ahlgrimm, J. Friemel, J. Kollmann, and H. Meilby, 2015: Growth response to climatic change over 120 years for Alnus viridis and Salix glauca in West Greenland. J. Vegetation Sci., 26, 155-165.
Kremers, K. S., R. D. Hollister, and S. F. Oberbauer, 2015: Diminished response of arctic plants to warming over time. PLOS One, 10, e0116586.
Myers-Smith, I. H., S. C. Elmendorf, P. S. A. Beck, M. Wilmking, M. Hallinger, D. Blok, K. D. Tape, S. A. Rayback, M. Macias-Fauria, B. C. Forbes, J. D. M. Speed, N. Boulanger-Lapointe, C. Rixen, E. Lévesque, N. Martin Schmidt, C. Baittinger, A. J. Trant, L. Hermanutz, L. Sieqwart Collier, M. A. Dawes, T. C. Lantz, S. Weijers, R. Halfdan Jørgensen, A. Buchwal, A. Buras, A. T. Naito, V. Ravolainen, G. Schaepman-Strub, J. A. Wheeler, S. Wipf, K. C. Guay, D. S. Hik, and M. Vellend, 2015: Climate sensitivity of shrub growth across the tundra biome. Nature Climate Change, doi:10.1038/nclimate2697.
Piao, S., H. Nan, C. Huntingford, P. Ciais, P. Friedlingstein, S. Sitch, S. Peng, A Ahlström, J. G. Canadell, N. Cong, S. Levis, P. E. Levy, L. Liu, M. R. Lomas, J. Mao, R. B. Myneni, P. Peylin, B. Poulter, S. Shi, G. Yin, N. Viovy, T. Wang, X. H. Wang, S. Zaehle, N. Zeng, Z. Z. Zeng, and A. P. Chen, 2014: Evidence for a weakening relationship between interannual temperature variability and northern vegetation activity. Nature Communications 5, 5018.
Preece, C., and G. K. Pheonix, 2014: Impact of early and late winter icing events on sub-arctic dwarf shrubs. Plant Biol., 15, 125-132.
Raynolds M. K., D. A. Walker, H. E. Epstein, J. E. Pinzon, and C. J. Tucker, 2012: A new estimate of tundra-biome phytomass from trans-Arctic field data and AVHRR NDVI. Remote Sens. Lett., 3, 403-411.
Ropars, P., E. Lévesque, and S. Boudreau, 2015: How do climate and topography influence greening of the forest-tundra ecotone in northern Québec? A dendrochronological analysis of Betula glandulosa. J. Ecology, 103, 679-690.
Sweet, S. K., K. L. Griffin, H. Steltzer, L. Gough, and N. T. Boelmann, 2015: Greater deciduous shrub abundance extends tundra peak season and increases modeled net CO2 uptake. Global Change Biol., doi:10.1111/gcb.12852.
van der Wal, R., and A. Stien, 2014: High-arctic plants like it hot: a long-term investigation of between-year variability in plant biomass. Ecology, 95, 3414-3427.
Zamin, T. J., M. S. Bret-Harte, and P. Grogan, 2014: Evergreen shrubs dominate responses to experimental summer warming and fertilization in Canadian mesic low arctic tundra. J. Ecology, 102, 749-766.
November 17, 2015