C. Derksen1, R. Brown1, L. Mudryk2, K. Luojus3
1Climate Research Division, Environment Canada, Toronto, Canada
2Department of Physics, University of Toronto, Canada
3Arctic Research Centre, Finnish Meteorological Institute, Helsinki, Finland
Highlights
- Arctic SCE anomalies in May and June 2015 (for land areas north of 60°N) were below the long-term average (1981-2010), a continuation of consistent early spring snow melt during the past decade. June SCE in both the North American and Eurasian sectors of the Arctic was the 2nd lowest in the satellite record (1967-present).
- For the fifth time in the past six years (2009-2015), total Arctic SCE in June was below 3 million km2, despite never falling below this threshold in the previous 43 years of the snow chart data record (1967-2008).
- The rate of June SCE reductions since 1979 (the start of the passive microwave satellite era used to monitor sea ice extent) is -17.2% per decade. Since 2011, the rate of June snow cover loss has exceeded the rate of September sea ice loss.
The Arctic (land areas north of 60°N) is always completely snow covered in winter, so it is the transition seasons of fall and spring that are significant when characterizing variability and change. The timing of spring snow melt is particularly important because the transition from highly reflective snow cover to the low albedo of snow-free ground is coupled with increasing solar radiation during the lengthening days of the high latitude spring. Strong snow-atmosphere feedbacks (including the well documented snow-albedo feedback), the energy sink induced by melting snow, and the strong thermal insulation effect of snow on the underlying soil all highlight the important role of variability and trends in Arctic snow cover extent (SCE) on the climate system and terrestrial ecosystems.
SCE anomalies (relative to the 1981-2010 reference period) for the 2015 Arctic spring (April, May, June) 2015 were computed separately for the North American and Eurasian sectors of the Arctic from the NOAA snow chart climate data record, maintained at Rutgers University (Estilow et al. 2015; http://climate.rutgers.edu/snowcover/). Consistent with nearly all spring seasons of the past decade, both May and June SCE anomalies were strongly negative in 2015 (Fig. 2.1); June SCE in both the North American and Eurasian sectors of the Arctic was the 2nd lowest in the snow chart record, which extends back to 1967.
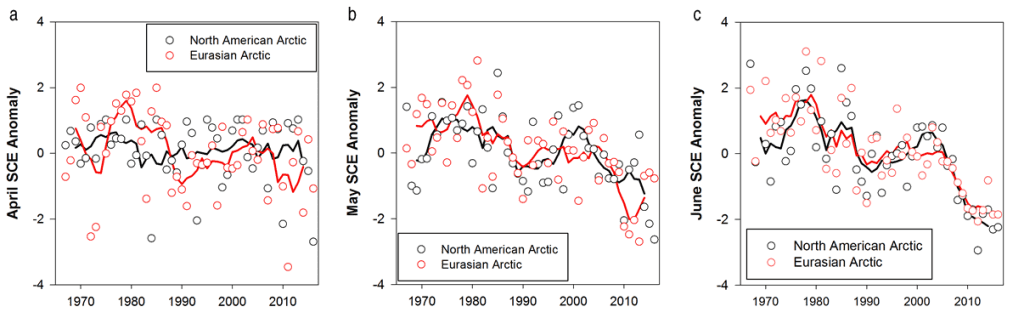
There are complex interactions between regional variability in the onset of snow cover in the autumn, subsequent winter season snow accumulation patterns (which themselves are driven by the complex interplay of temperature and precipitation anomalies) and the continental scale spring SCE anomalies (Fig. 2.1). Snow cover duration (SCD) departures derived from the NOAA daily Interactive Multisensor Snow and Ice Mapping System (IMS) snow cover product (Helfrich et al. 2007) show earlier snow cover onset in the fall over much of the Arctic, for the 2014-2015 snow year (Fig. 2.2a). This is consistent with pre-melt snow depth anomalies (derived from the Canadian Meteorological Centre daily gridded global snow depth analysis; Brasnett 1999), which were largely positive over much of the Arctic land surface (+25.1% and +33.7%, respectively, for the North American and Eurasian sectors of the Arctic) (Fig. 2.3b). There was a notable east-west snow depth gradient across Eurasia with above average snow depth in eastern Siberia and below average snow depth across western Siberia and northern Europe. The North American Arctic was characterized by a more latitudinal gradient of deeper than normal snow depth north of the boreal tree line and shallower than normal snow depth across the boreal forest (Figs. 2.3a and 2.3b). Note that the CMC results shown in Fig. 3 mask out anomalies over high elevation areas (in the Canadian Arctic Archipelago, Baffin Island, coastal Alaska) known to be affected by an internal trend of higher winter snow depths since 2006 due to changes in the resolution of the precipitation forcing used as part of the CMC analysis.
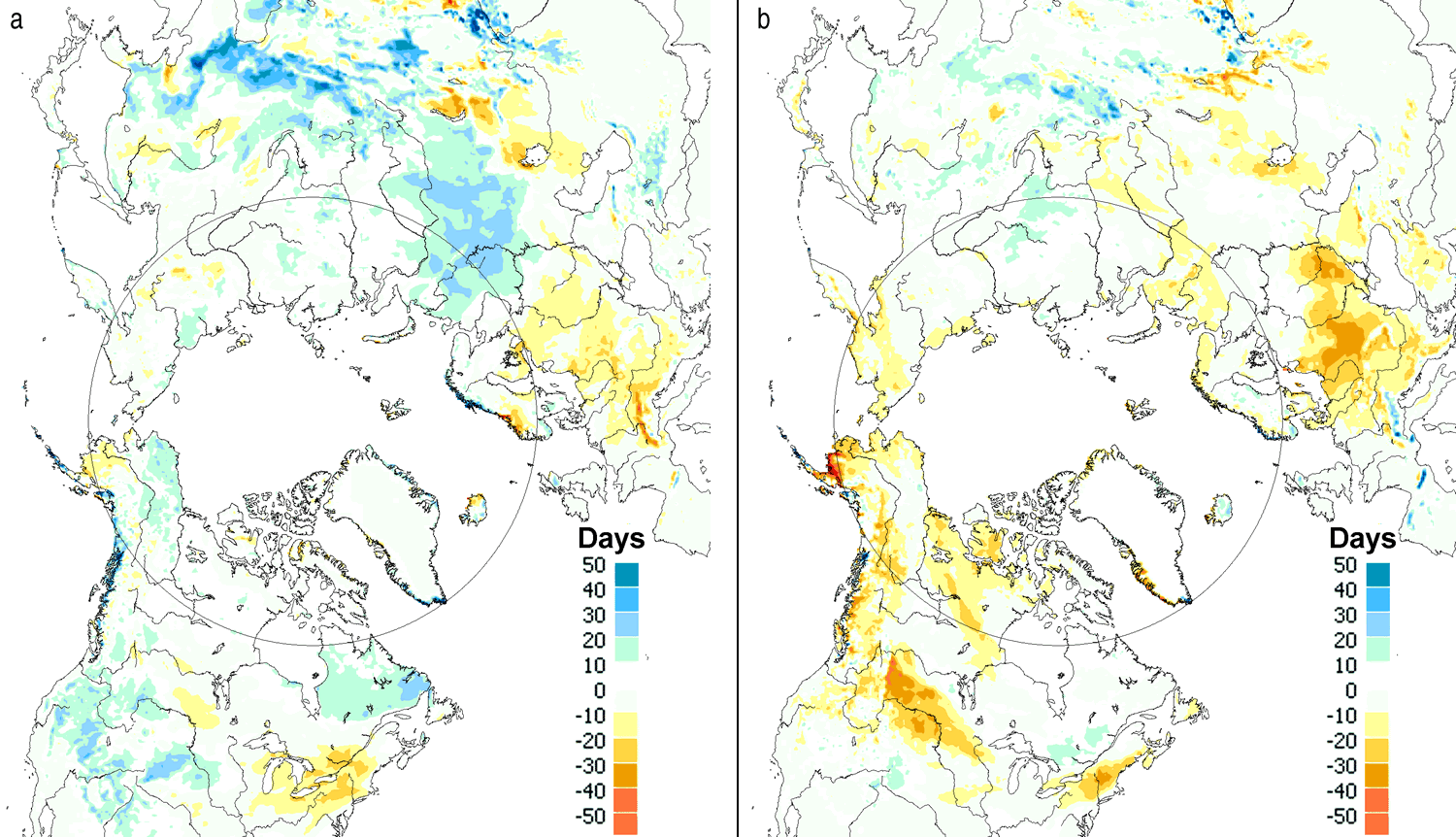
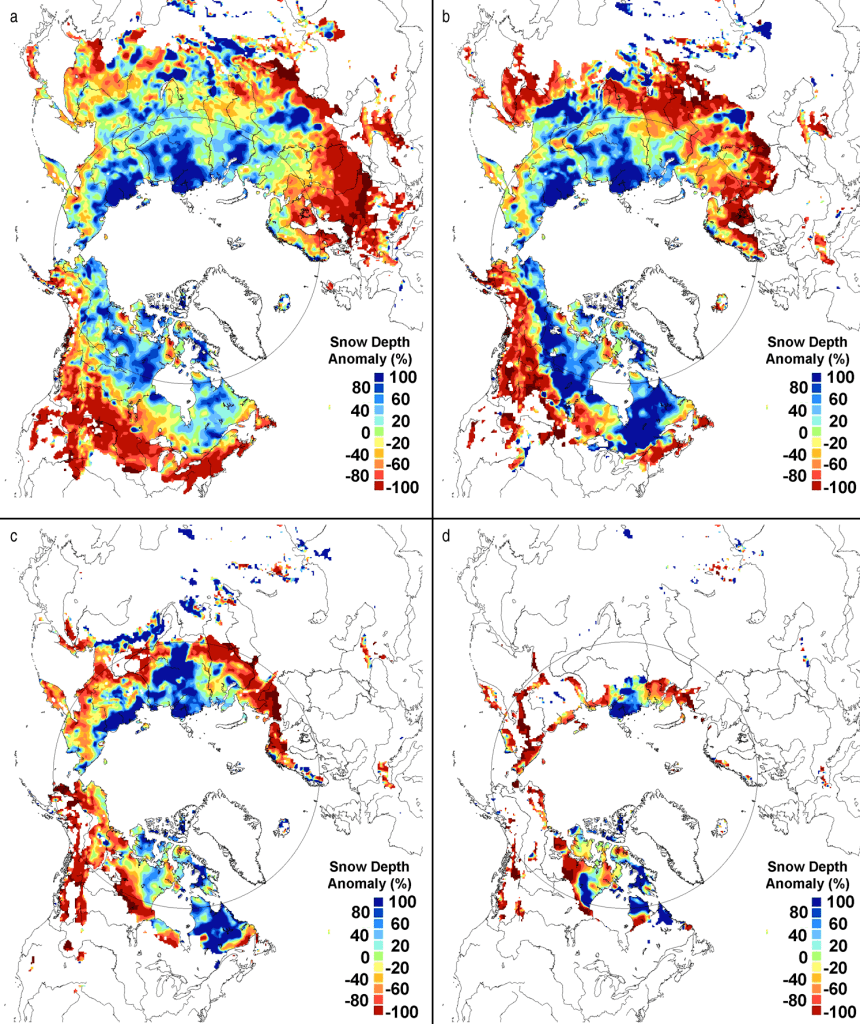
Strong positive surface temperature anomalies over central Siberia, Alaska and the western Canadian Arctic in May (which persisted into June; see Fig. 1.2c in the essay on Air Temperature) were associated with rapid reductions in regional snow depth (Figs. 2.3c and 2.3d) and earlier than normal snow melt in these regions (Fig. 2.2b), which drove the negative continental-scale SCE anomalies in May and June. For the fifth time in the past six years (2009-2015), Arctic SCE in June was below 3 million km2 (Fig. 2.4a) despite never falling below this threshold in the previous 43 years of the snow chart data record (1967-2008). Figure 2.4 shows the changing rate of SCE loss across the Arctic since 1998 via calculations over running time periods since 1979, the first year of the satellite passive microwave record used to track sea ice extent (calculations were made for 1979-1998, 1979-1999, 1979-2000, and so on). The April and May SCE reductions have remained relatively consistent year over year, ranging between -1% and -2% per decade (April) and -3% and -5% per decade (May). In contrast, since 2005, the rate of June SCE loss has increased from approximately -8% per decade (1979-2005) to -17% per decade (1979-2015). Since 2011, the rate of June snow cover loss has exceeded the rate of September sea ice loss.
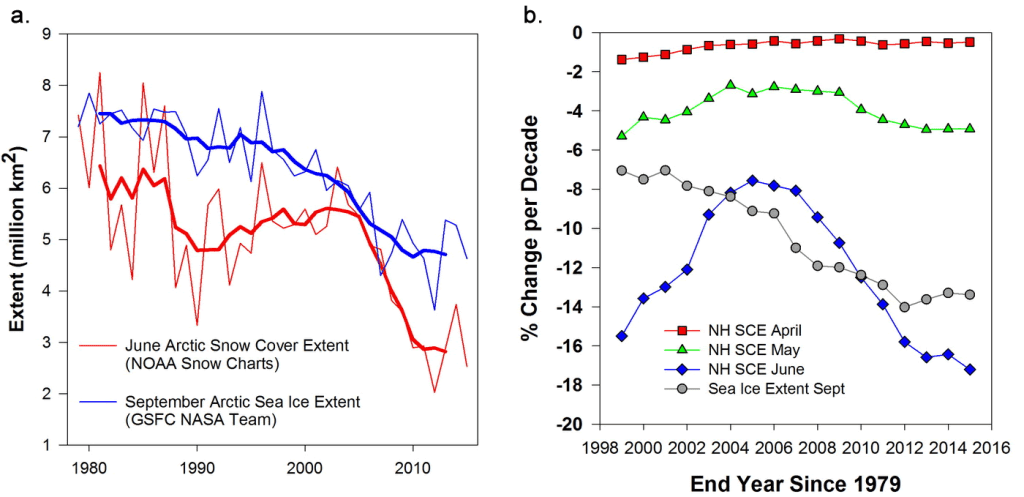
The 2015 spring melt season provided continued evidence of earlier snowmelt across the terrestrial Arctic. There is increased awareness of the impact of these changes on the cryosphere and the Arctic environment (Callaghan et al. 2013). There remains a need to better quantify and understand the impacts of variability and trends in high latitude snow cover in the fall season, given the potential remote impacts on the climate system (Cohen et al. 2014) but higher observational uncertainty during this time of year because of a short daylight period and increases in cloud cover that accompany snow cover onset (Brown and Derksen 2013).
References
Brasnett, B., 1999: A global analysis of snow depth for numerical weather prediction. J. Appl. Meteorol., 38, 726-740.
Brown, R., and C. Derksen, 2013: Is Eurasian October snow cover extent increasing? Env. Res. Lett., 8, 024006 doi:10.1088/1748-9326/8/2/024006.
Callaghan, T., M. Johansson, R. Brown, P. Groisman, N. Labba, V. Radionov, R. Barry, O. Bulygina, R. Essery, D Frolov, V. Golubev, T. Grenfell, M. Petrushina, V. Razuvaev, D. Robinson, P. Romanov, D. Shindell, A. Shmakin, S. Sokratov, S. Warren, D. Yang, 2011: The changing face of Arctic snow cover: A synthesis of observed and projected changes. Ambio, 40:17-31.
Cavalieri, D. J., C. L. Parkinson, P. Gloersen, and H. J. Zwally, 1996, updated yearly: Sea Ice Concentrations from Nimbus-7 SMMR and DMSP SSM/I-SSMIS Passive Microwave Data, Version 1 [1979-2014]. Boulder, Colorado USA. NASA National Snow and Ice Data Center Distributed Active Archive Center. http://dx.doi.org/10.5067/8GQ8LZQVL0VL.
Cohen, J., J. Furtado, J. Jones, M. Barlow, D. Whittleson, and D. Enekhabi, 2014: Linking Siberian Snow Cover to Precursors of Stratospheric Variability. J. Climate, 27: 5422-5432.
Estilow, T. W., A. H. Young, and D. A. Robinson, 2015: A long-term Northern Hemisphere snow cover extent data record for climate studies and monitoring. Earth Sys. Sci. Data, 7.1: 137-142.
Helfrich, S., D. McNamara, B. Ramsay, T. Baldwin, and T. Kasheta, 2007: Enhancements to, and forthcoming developments in the Interactive Multisensor Snow and Ice Mapping System (IMS). Hydrol. Process., 21, 1576-1586.
Maslanik, J. and J. Stroeve, 1999, updated daily: Near-Real-Time DMSP SSMIS Daily Polar Gridded Sea Ice Concentrations, Version 1. [September 2015]. Boulder, Colorado USA. NASA National Snow and Ice Data Center Distributed Active Archive Center. http://dx.doi.org/10.5067/U8C09DWVX9LM.
November 17, 2015