A. G. Hope1, S. E. Greiman2, V. V. Tkach3, E. P. Hoberg4, J. A. Cook5
1Division of Biology, Kansas State University, Manhattan, KS, USA
2Department of Biology, Georgia Southern University, Statesboro, GA, USA
3Department of Biology, University of North Dakota, Grand Forks, ND, USA
4US Department of Agriculture, Agricultural Research Service, Beltsville, MD, USA
5Department of Biology and Museum of Southwestern Biology, University of New Mexico, Albuquerque, NM, USA
Highlights
- As Arctic climate warms, how will terrestrial ecosystems and interconnected communities respond in the coming decades? Small mammals, such as shrews, and their parasites can serve as key indicators for anticipating the consequences of accelerating environmental perturbation and changing interactions among species.
- Some Arctic shrews have recently acquired new parasites indicating poleward shifts of sub-Arctic faunas, and demonstrating increases in both Arctic biodiversity and overall complexity within these novel species networks.
- Spatially broad and temporally deep specimen collections of small mammals and parasites are revealing the extent and nature of community reorganization as northern faunas adjust to accelerating climate change.
Introduction
When natural environments change, species may shift their distributions, adapt to novel conditions, or die out. Consequently, accelerating climate change is already recognized as leading to considerable range expansion and contraction, evolutionary changes, and extinction for hosts, parasites, and diseases through the Arctic (e.g., Kutz et al., 2013; Meltofte et al., 2013). Ultimately, these perturbations have consequences for wildlife and humans at high latitudes (e.g., Dudley et al., 2015). Complex host-parasite systems are critical proxies for understanding change in northern regions due to species interactions that determine the distribution of parasites and disease over space and time (e.g., Hoberg et al., 2012, 2013). Life histories for helminth parasites (tapeworms, flukes, roundworms) often involve circulation among mammalian hosts (where adult parasites reside) and other vertebrate and invertebrate species (where larval or developing parasites reside). Other parasites (viruses, bacteria, protozoans) circulate through vectors such as blood feeding arthropods or through direct transmission. These complex life cycles are closely tied to environmental conditions, define linkages across communities, and scale from individuals to ecosystems.
Small mammals, and especially shrews (Sorex spp.; Fig. 10.1), are diverse and relatively well documented through the Arctic (MacDonald and Cook, 2009). Shrews occupy most habitats, host a diverse and abundant parasite fauna, and function as consumers of considerable quantities of invertebrates, widely ranging from worms and crustaceans, to spiders, insects, and their larvae. Because of these connections, shrews and parasites provide direct insights into processes governing larger networks of organisms within terrestrial ecosystems (Binkienė et al., 2011; Hope et al., 2013). From shrew faunas, we now recognize how climate change through ice ages of the past two million years has facilitated repeated invasions of northern systems (e.g., Hope et al., 2015), movement between continents (Waltari et al., 2007), contact among other mammalian or avian species including exchange of parasites (Haukisalmi et al., 2010), and at times extended isolation in glacial refugia (Cook et al. 2005). We review recent advances in our understanding of the complexity of these small mammals and their parasites, emphasizing their value for interpreting generalized biodiversity responses to accelerating change and perturbation in the Arctic.
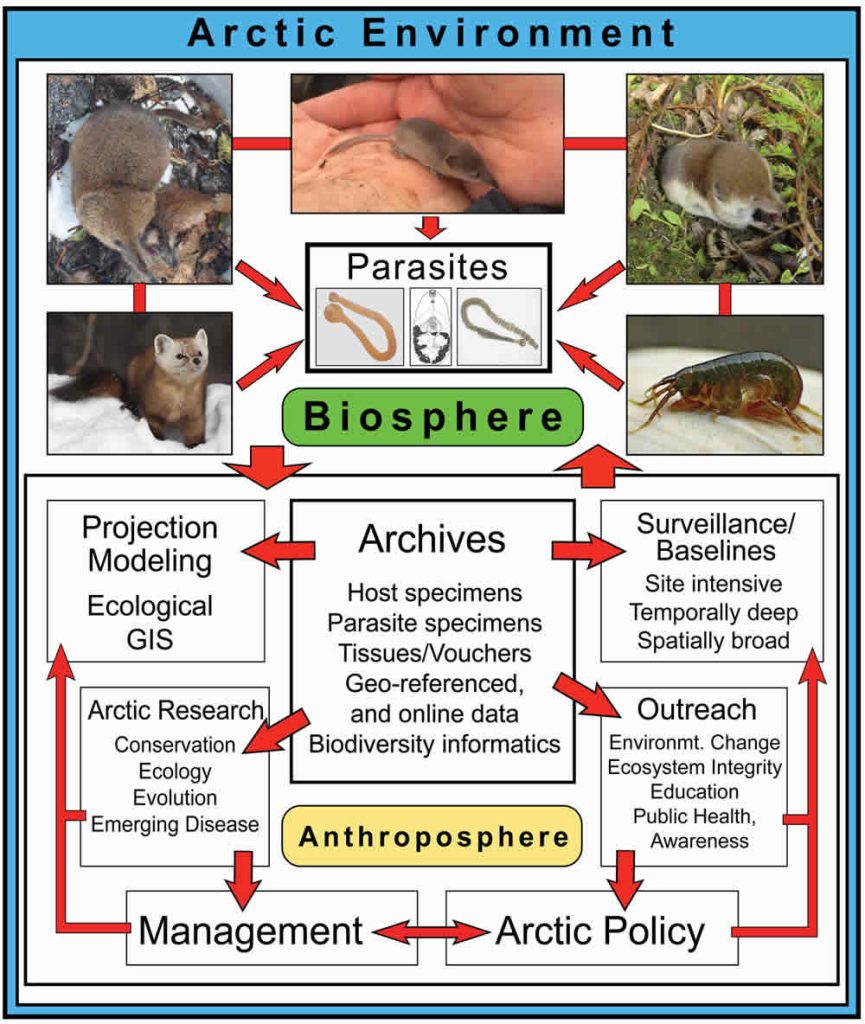
Shrews and Parasites Reflect Generalizable Processes
Research on shrews and their parasites aims to mitigate future loss of Arctic biodiversity due to anthropogenic environmental change, habitat alteration, and novel species interactions. Persistent challenges for conservation of Arctic biodiversity include incomplete knowledge of 1) existing faunal diversity and structure, 2) ecological processes that maintain critical connections among species, and 3) processes that drive evolution (diversification and adaptation) within wild populations (Dobson et al., 2015).
Most shrew species exhibit broad continental distributions, with regional variation corresponding to major geographic barriers (e.g., Hope et al., 2012). As shrews are well represented in northern communities and are conducive to detailed specimen-based analyses, we use them to understand fundamental evolutionary and ecological processes that govern all species, from mites to muskox (Hoberg and Brooks, 2008). Genetic signatures recorded in shrew DNA can be used to understand past distributions, population sizes, and responses to environmental change. Long-term population characteristics can then be combined with climate data from the last 30 years to understand recent distributions and to project trajectories into future decades. For example, species distributional models for masked shrews (Sorex cinereus) associated with forests and barren ground shrews (Sorex ugyunak) associated with tundra indicate that the latter species is experiencing significant range contraction and fragmentation in multiple refugial areas, whereas populations of the former are expanding their distributions on northward trajectories (Fig. 10.2); trends that are predicted to continue into the near future.


Coupling genetic data (recording past responses) with climate data (governing present and future distributions) provides a powerful toolset for gauging the rate, extent, and direction of shrew responses to climate change. Our analyses indicate that Arctic adapted shrews occurring in tundra have persisted within northernmost regions through extended cold periods, often in the absence of sub-Arctic species, and with more extensive distributions than at present (Fig. 10.3; Hope et al., 2015). As climate has transitioned from cold to warm, our models indicate progressive contraction and fragmentation into future refugial areas of intact tundra biodiversity that are nested within historical distributions for this fauna. These refugial zones are significant in reflecting areas of continuous occupation, and a long history of ebb and flow within Arctic ecosystems. Conversely, multiple shrews species associated with boreal (forest) habitats did not endure within Arctic latitudes through cold phases of the Pleistocene. These species have rapidly shifted ranges northward with climate warming to follow their preferred set of environmental conditions, a trend that will continue in the coming decades (Hope et al., 2013). Differential responses among forest and tundra species have resulted in range overlap and increased complexity of species interactions (Hoberg and Brooks, 2013). Support for observed shrew responses are strengthened by analysis of other mammal species, indicating concerted community-level outcomes involving northward expansion into the Arctic among forest species, and northward contraction and fragmentation of Arctic species (Fig. 10.3; Hoberg et al., 2012; Hope et al., 2014, 2015).
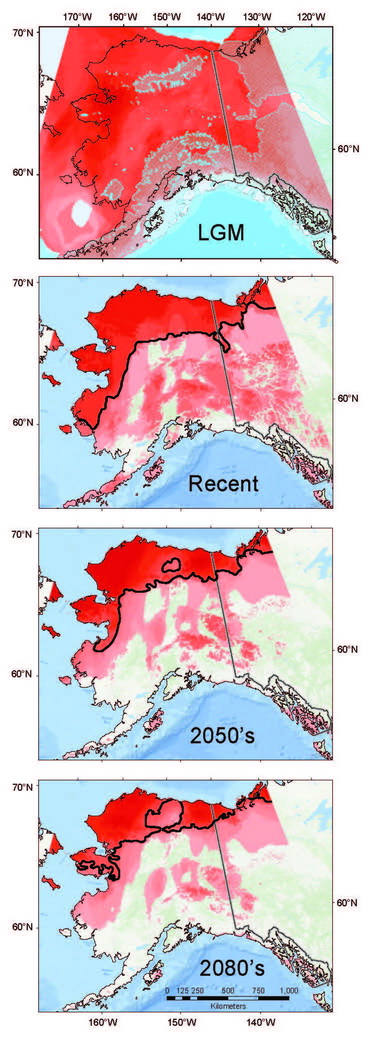
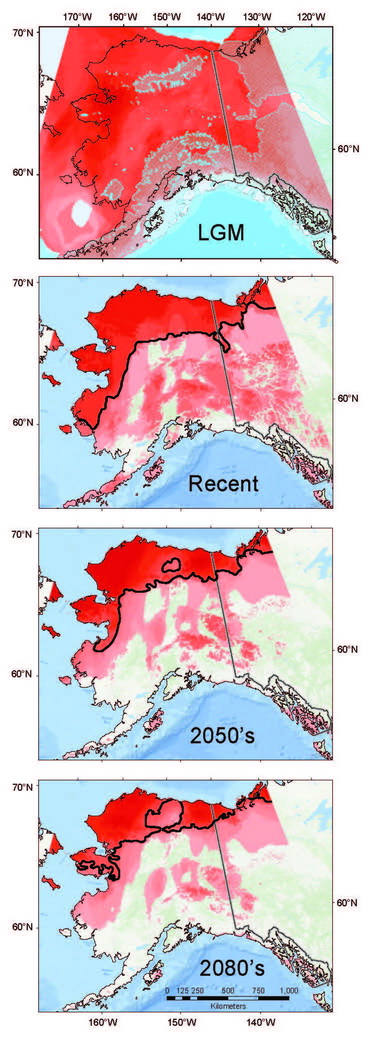
Shrew parasites variably adhere to host responses through complex interactions which involve 1) geographic colonization, particularly intercontinental exchange, 2) host switching, and 3) local evolutionary adaptation to changing environments (e.g., Hoberg and Brooks, 2015). Future viability of Arctic biodiversity will be contingent on sensitivity to environmental thresholds, and relative ability to persist in the face of change, reflecting both resilience and ecological plasticity (Araujo et al., 2015). As such, although many Arctic taxa have persisted until now (through major pre-Anthropocene climate oscillations), they may be particularly vulnerable to a contemporary regime of accelerated warming not previously experienced (Serreze and Barry, 2011). Overlap among previously isolated species will create new associations which can be more readily examined within shrews and their diverse complement of parasites, compared with larger species (e.g., caribou). Hybridization between closely related species may play a critical role in diversification, adaptation, or even extinction (Hewitt, 2011). The current overlap between boreal and tundra communities in Alaska occurs in Low-Arctic tundra, where we have recently identified hybridization between shrews in these boundary zones (Hope et al., In Prep.). Secondary contact and host hybridization also undoubtedly contributes to mixing among parasites, intensifying these dynamics. The interplay between shrew hybridization and mixing of parasite communities result in faunal mosaics that are now a critical component being explored within a broader research agenda in the Arctic, where diverse species assemblages are undergoing complex patterns of exchange (Kelly et al., 2010; Hoberg et al., 2012).
An exemplar of shrew-parasite dynamics is being revealed within a remote island system. Islands typically have fewer species with limited ranges, lower genetic variability and reduced ability to respond to change (Pauls et al., 2013). For example, the remote island of St. Paul was isolated in the Bering Strait about 10,000 years ago and supports only a single small mammal species, the Pribilof Islands shrew (Sorex pribilofensis). Through collaborations with Aleuts of St. Paul and NOAA, we obtained samples of S. pribilofensis and confirmed that they have extremely low genetic diversity and a reduced parasite fauna dominated by a tapeworm (Lineolepis pribilofensis), which occurs in great abundance (sometimes hundreds per shrew).
How mammals regulate their parasites, or what conditions allow them to host diverse versus more limited parasite faunas is contingent on history and ecology, and in turn parasite diversity or intensity of infection may regulate gut microbiomes, including pathogens (Bordes and Morand, 2015). Notably, some S. pribilofensis specimens, in addition to being infected with a single shrew-specific tapeworm, also had heavy loads of Maritrema flukes. Successful infection by these helminths in shrews is remarkable considering their typical hosts are shorebirds, with parasite life cycles occurring in the intertidal zone as birds (and shrews) eat crustacean intermediate hosts such as amphipods (Fig. 10.4). This is an intriguing example of how island populations contribute to intercontinental transfer of parasites both between distantly related avian and mammalian hosts and through marine to terrestrial pathways via intertidal foraging, as seen elsewhere (Stewart et al., 1989).
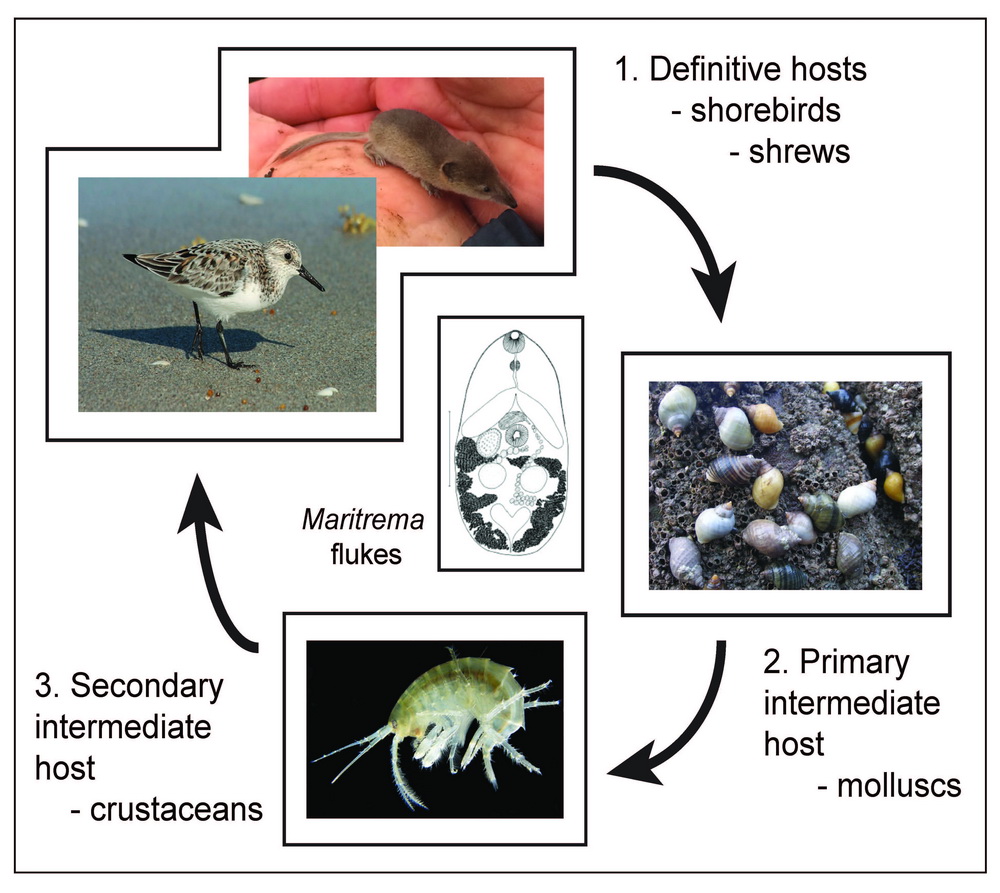
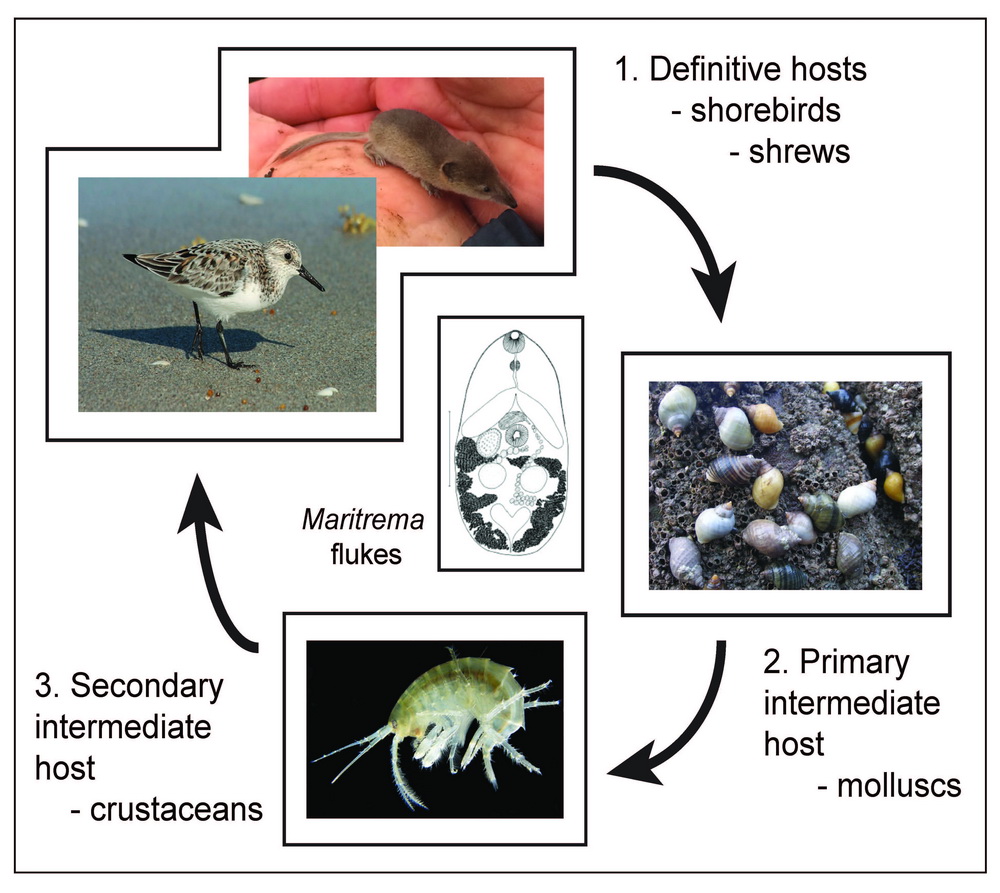
St. Paul Island is coincident with a transition zone between the Arctic and Pacific oceans, and provides another arena for complex species interactions at the intersection between marine and terrestrial ecosystems. As climate in the Bering Sea warms, this marine transition zone has shifted northward to the vicinity of St. Paul, disrupting the ecological isolation of purely Arctic communities in this area and increasing the diversity of littoral species, including amphipods (Mueter and Litzow, 2008; Weslawski et al., 2010). This shift may explain why Maritrema flukes now infect S. pribilofensis, but did not only ~50 years ago (Olsen, 1969). Such cascading effects highlight conservation implications for island species with nowhere to go, low potential to adapt, and which are now experiencing new relationships with potential pathogens; all consequences of a warming climate, ecosystems in rapid transition, and a shrinking Arctic (e.g., Hoberg et al., 2013).
Historical Archives Fuel Future Understanding
A primary issue for anticipating responses within high latitude systems continues to be a woefully incomplete knowledge of existing Arctic biodiversity (e.g., MacDonald and Cook, 2009; Hoberg et al., 2013; Cook et al., 2013). Recent compilations of shrew endoparasites (Kinsella and Tkach, 2009; Binkienė et al., 2011) highlights large gaps in our understanding of northern parasites and also documents high rates of new discoveries of parasites and viruses (Arai et al., 2008; Greiman et al., 2013; Tkach et al., 2013). However, new efforts to develop site intensive and spatially broad natural history collections are providing a rigorous foundation for multiple integrative approaches to characterize diversity and understand changing conditions (Fig. 10.1; McLean et al., 2015; Hoberg et al., 2015), including recognition of new shrew species and new distributional records (Cook et al., 2016). For example, genomic sequencing of historic and recent specimens is providing unparalleled views of contemporary population responses, parasite and microbiome biodiversity, and changing Arctic conditions (Cook et al., in review).
Current rates of Arctic environmental perturbations substantially surpass global averages (Serreze and Barry, 2011) while our ability to comprehensively understand the impacts for humans and intact wild systems continues to scratch the surface (Meltofte et al., 2013). A robust picture of diversity in the Arctic urgently requires coordinated efforts to synthesize traditional knowledge and insights from indigenous peoples, along with implementation of integrated and comprehensive field collections. An emphasis on annual targeted monitoring will provide both archival specimens and ecological information as baselines to anticipate regional and landscape responses to accelerating change.
Host-parasite systems provide a generalizable reference for understanding and documenting ecological changes (e.g., Kutz et al., 2014). Utilizing diverse, widespread, and abundant host/parasite resources from both small and large mammals, and associated communities affords critical insights about the nature and future of high latitude systems (Hoberg et al., 2012, 2013; Hope et al., 2013). As northern species have developed new associations with observable frequency through recent decades, we anticipate increasing occurrence of host switching events (and potential for emerging disease). Dynamic faunas such as shrews and their parasites embody the concept of “communities within communities” and are revealing the complexity of evolutionary and ecological processes now shaping Arctic terrestrial ecosystems.
References
Arai, S., S. N. Bennett, L. Sumibcay, J. A. Cook, J. W. Song, A. Hope, C. Parmenter, V. R. Nerurkar, T. L. Yates, and R. Yanagihara, 2008: Phylogenetically distinct hantaviruses in the masked shrew (Sorex cinereus) and dusky shrew (Sorex monticolus) in the United States. Am. J. Trop. Med. Hyg., 78, 348-351.
Araujo, S. B. L., M. P. Braga, D. R. Brooks, S. Agosta, E. P. Hoberg, F. von Hathental, W. A. Boeger, 2015: Understanding host-switching by ecological fitting. PLoS ONE, 10, e0139225.
Binkienė, R., V. Kontrimavichus, and E. Hoberg. 2011: Overview of the cestode fauna of European shrews of the genus Sorex with comments on the fauna in Neomys and Crocidura and an exploration of historical processes in post-glacial Europe. Helminthologia, 48, 207-228.
Bordes, F., and S. Morand, 2015: Impacts of parasite diversity on wild vertebrates: limited knowledge but important perspectives, Ch. 5. In: (Eds. S. Morand, B. R. Krasnov, and D. T. L. Littlewood) Parasite Diversity and Diversification: Evolutionary Ecology Meets Phylogenetics, Cambridge University Press, Cambridge, United Kingdom.
Cook, J. A., E. P. Hoberg, A. Koehler, H. Henttonen, L. Wickström, V. Haukisalmi, K. Galbreath, F. Chernyavski, N. Dokuchaev, A. Lahzuhtkin, S. O. MacDonald, A. Hope, E. Waltari, A. Runck, A. Veitch, R. Popko, E. Jenkins, S. Kutz, and R. Eckerlin, 2005: Beringia: intercontinental exchange and diversification of high latitude mammals and their parasites during the Pliocene and Quaternary. Mamm. Study, 30, S33-S44.
Cook, J., C. Brochman, S. L. Talbot, V. Fedorov, E. Taylor, R. Väinölä, E. P. Hoberg, M. Kholodova, M. Magnusun, 2013: Genetics, pp. 514-539 In: (Ed. H. Meltofte) Arctic Biodiversity Assessment- Status and Trends in Arctic Biodiversity. Conservation of Arctic Flora and Fauna, Arctic Council, Akureyi, Iceland.
Cook, J. A., B. S. McLean, D. J. Jackson, J. P. Colella, S. E. Greiman, V. V. Tkach, T. S. Jung, and J. L. Dunnum, 2016: First record of the Holarctic least shrew (Sorex minutissimus) and associated helminths from Canada: new light on northern Pleistocene refugia. Can. J. Zool., 94, 367-372.
Dobson, A., P. K. Molnár, and S. Kutz, 2015: Climate change and Arctic parasites. Trend. Parasitol., 31, 181-188.
Dudley, J. P., E. P. Hoberg, E. J. Jenkins, A. J. Parkinson, 2015: Climate change in the North American Arctic: A One Health perspective. EcoHealth, 12, 713-725.
Greiman, S. E., V. V. Tkach, and J. A. Cook, 2013: Description and molecular differentiation of a new Staphylocystoides (Cyclophyllidea: Hymenolepididae) from the dusky shrew Sorex monticolus in Southeast Alaska. J. Parasitol. 99, 1045-1049.
Haukisalmi, V., L. M. Hardman, P. Foronda, C. Feliu, J. Laakkonen, J. Niemimaa, J. T. Lehtonen, and H. Henttonen, 2010: Systematic relationships of hymenolepidid cestodes of rodents and shrews inferred from sequences of 28S ribosomal RNA. Zoologica Scripta, 39, 631-641.
Hewitt, G. M., 2011: Quaternary phylogeography: the roots of hybrid zones. Genetica, 139, 617-638.
Hoberg, E. P., and D. R. Brooks, 2008: A macroevolutionary mosaic: Episodic host-switching, geographic colonization, and diversification in complex host-parasite systems. J. Biogeog., 35, 1533-1550.
Hoberg, E. P., and D. R. Brooks, 2013: Episodic processes, invasion, and faunal mosaics in evolutionary and ecological time, pp. 199-213 In: (Ed. K. Rohde) The Balance of Nature and Human Impact. Cambridge University Press.
Hoberg, E. P., and D. R. Brooks, 2015: Evolution in action: Climate change, biodiversity dynamics and emerging infectious disease. Theme Issue- Climate Change and Vector-Borne Diseases. Phil. Trans. R. Soc. B, 2015: 370, 20130553.
Hoberg, E. P., K. E. Galbreath, J. A. Cook, S. J. Kutz, and L. Polley, 2012: Northern host-parasite assemblages: History and biogeography on the borderlands of episodic climate and environmental transition, pp. 1-97 In: (Eds. D. Rollinson and S.I. Hays) Advances in Parasitology, 79, Elsevier.
Hoberg, E. P., S. J. Kutz, J. A. Cook, K. Galaktionov, V. Haukisalmi, H. Henttonen, S. Laaksonen, A. Makarikov, and D. J. Marcogliese, 2013: Parasites in terrestrial, freshwater and marine systems, pp. 476-505 In: (Ed. H. Meltofte) Arctic Biodiversity Assessment- Status and Trends in Arctic Biodiversity. Conservation of Arctic Flora and Fauna, Arctic Council, Akureyi, Iceland.
Hoberg, E. P., S. J. Agosta, W. A. Boeger, and D. R. Brooks, 2015: An Integrated parasitology: Revealing the elephant through tradition and invention. Trend. Parasitol., 31, 128-133.
Hope, A. G., K. A. Speer, J. R. Demboski, S. L. Talbot, and J. A. Cook, 2012. A climate for speciation: Rapid spatial diversification within the Sorex cinereus complex of shrews. Mol. Phylogenet. Evol., 64, 671-684.
Hope, A. G., E. Waltari, D. C. Payer, J. A. Cook, and S. L. Talbot, 2013: Future distribution of tundra refugia in northern Alaska. Nature Clim. Change, 3, 931-938.
Hope, A. G., S. W. Y. Ho, J. L. Malaney, J. A. Cook, and S. L. Talbot, 2014a: Accounting for rate variation among lineages in comparative demographic analyses. Evolution, 68, 2689-2700.
Hope, A. G., E. Waltari, J. L. Malaney, D. C. Payer, J. A. Cook, and S. L. Talbot, 2015. Arctic biodiversity: increasing richness accompanies shrinking refugia for a cold-associated tundra fauna. Ecosphere, 6, 1-67.
Karpenko, C. V., and N. E. Dokuchaev, 2012. The first record of the trematode Maritrema afanassjewi Belopolskaja, 1952 in shrews. Vestnik Dalnevostochnogo Otdeleniya Rossiyskoj Akademii Nauk, 163, 103-106. (in Russian)
Kelly, B., D. A. Tallmon, and A. Whiteley, 2010: The Arctic melting pot. Nature, 468, 891.
Kinsella, J. M., and V. V. Tkach, 2009: Checklist of helminth parasites of Soricomorpha (= Insectivora) of North America north of Mexico. Zootaxa, 1969, 36-58.
Kutz, S. J., S. Checkley, G. G. Verocai, M. Dumond, E. P. Hoberg, R. Peacock, J. Wu, K. Orsel, K. Seegers, A. Warren, and A. Abrams, 2013: Invasion, establishment, and range expansion of two parasitic nematodes in the Canadian Arctic. Global Change Biol., 19, 3254-3262.
Kutz, S., E. P. Hoberg, P. K. Molnár, A. Dobson, and G. Verocai, 2014: A walk on the tundra: Host-parasite interactions in an extreme environment. Internat. J. Parasitol.- Parasites Wildlife, 3, 198-208.
MacDonald, S. O. and J. A. Cook, 2009: Recent Mammals of Alaska. University of Alaska Press, Fairbanks. 387pp.
McLean, B. S., K. C. Bell, J. L. Dunnum, B. Abrahamson, J. P. Colella, E. R. Deardorff, J. A. Weber, A. K. Jones, F. Salazar-Miralles, and J. A. Cook, 2015: Natural history collections-based research: progress, promise, and best practices. J. Mamm., p. 178.
Meltofte, H., T. Barry, D. Berteaux, H. Bultmann, J. S. Christiansen, J. A. Cook, A. Dahlberg, F. J. A. Daniëls, D. Ehrich, J. Fjeldså, F. Fridriksson, B. Ganter, A. J. Gaston, L. J. Gillespie, L. Grenoble, E. P. Hoberg, I. D. Hodkinson, H. P. Huntington, R. A. Ims, A. B. Josefson, S. J. Kutz, S. L. Kuzmin, K. L. Laidre, D. R. Lassuy, P. N. Lewis, C. Lovejoy, C. Michel, V. Mokievsky, T. Mustonen, D. C. Payer, M. Poulin, D. G. Reid, J. D. Reist, D. F. Tessler, and F. J. Wrona, 2013: Arctic Biodiversity Assessment. Conservation of Arctic Fauna and Flora Committee, Copenhagen.
Mueter, F. J. and M. A. Litzow, 2008: Sea ice retreat alters the biogeography of the Bering Sea continental shelf. Ecol. App., 18, 309-320.
Olsen, O. W., 1969: Hymenolepis pribilofensis n. sp. (Cestoda: Hymenolepididae) from the Pribilof shrew (Sorex pribilofensis Merriam) from the Pribilof Islands, Alaska. Can. J. Zool., 47, 449-454.
Pauls, S. U., C. Nowak, M. Bálint, and M. Pfenninger, 2013: The impact of global climate change on genetic diversity within populations and species. Mol. Ecol., 22, 925-946.
Serreze, M. C. and R. G. Barry, 2011. Processes and impacts of Arctic amplification: A research synthesis. Glob. Planet. Change, 77, 85-96.
Stewart, D. T., T. B. Herman, and T. Teferi, 1989. Littoral feeding in a high-density insular population of Sorex cinereus. Can. J. Zool., 67, 2074-2077.
Tkach, V. V., A. A. Makarikov, and J. M. Kinsella, 2013: Morphological and molecular differentiation of Staphylocystis clydesengeri n. sp. (Cestoda, Hymenolepididae) from the vagrant shrew, Sorex vagrans (Soricimorpha, Soricidae) in North America. Zootaxa, 3691, 389-400.
Waltari, E., E. P. Hoberg, E. P. Lessa, and J. A. Cook, 2007: Eastward Ho: phylogeographical perspectives on colonization of hosts and parasites across the Beringian nexus. J. Biogeog., 34, 561-574.
Weslawski J. M., J. Wiktor Jr., and L. Kotwicki, 2010: Increase in biodiversity in the arctic rocky littoral, Sorkappland, Svalbard, after 20 years of climate warming. Marine Biodiv., 40, 123-130.
December 12, 2016