L. R. Mudryk1, A. Elias Chereque2, C. Derksen1, K. Luojus3, and B. Decharme4
1Climate Research Division, Environment and Climate Change Canada, Toronto, ON, Canada
2Department of Physics, University of Toronto, Toronto, ON, Canada
3Arctic Research Centre, Finnish Meteorological Institute, Helsinki, Finland
4Centre National de Recherches Météorologiques, Météo-France, Toulouse, France
Headlines
- Snow accumulation during the 2023/24 winter was above the 1991-2020 average across both the Eurasian and North American Arctic.
- Early snow onset and delayed spring melt resulted in a longer than average snow season over much of the Eurasian Arctic.
- Over North America late snow onset and early spring melt resulted in the shortest snow season seen in 26 years over portions of central and eastern Arctic Canada.
- Compared to historical conditions, Arctic snow melt over the past 15 years has commonly occurred 1-2 weeks earlier throughout May and June over both Eurasia and North America.
Introduction
Many Arctic land surface processes are directly influenced by snow cover from autumn through spring, including the surface energy budget, ground temperature regime, permafrost, and terrestrial and freshwater ecosystems (Brown et al. 2017; Meredith et al. 2019). Even following the snow cover season, the influence of spring snow melt timing persists through impacts on river discharge timing and magnitude, surface water, soil moisture, vegetation phenology, and fire risk (Meredith et al. 2019).
Multiple data sets derived from satellite observations and snowpack models driven by atmospheric reanalyses are used to assess Arctic seasonal snow cover (see Methods and data). Collectively, this approach provides a reliable picture of Arctic snow cover variability over the last five decades. We characterize snow conditions across the Arctic land surface using three quantities: how much total land area is covered by snow (snow cover extent – SCE), how much of the year snow covers the land surface (snow cover duration – SCD), and how much total water is stored in solid form by the snowpack (snow water equivalent – SWE; the product of snow depth and density and which when aggregated over a region measures the mass of snow). We examine each of these quantities in turn for the 2023/24 Arctic snow season.
Snow cover extent and duration
SCE anomalies (relative to the 1991-2020 baseline) in spring 2024 are shown separately for the North American and Eurasian sectors of the Arctic in Fig. 1. Eurasian Arctic SCE was close to normal or slightly above normal during May (20th lowest May SCE in the 58-year record) related to cold spring conditions over northern Europe and western Russia (see essay Surface Air Temperature). By June, however, Eurasian Arctic SCE dropped below normal (14th lowest June SCE in the 58-year record). This change from above to below normal snow extent between May and June is clear from weekly anomalies (Fig. 2) whereby a sharp transition from above normal to below normal snow extent occurred between weeks 20 and 22 of the year (end of May). Corresponding SCD anomalies for the 2023/24 snow season (Fig. 3) indicate primarily early onset of snowfall and delayed melt during the spring across broad portions of northern Europe, western Russia, and easternmost Siberia (relative to a 25-year baseline from 1998/99 to 2022/23). The combination of early onset and delayed melt led to one of the longer snow seasons of the past 26 years across the majority of the Eurasian Arctic (Fig. 3c).
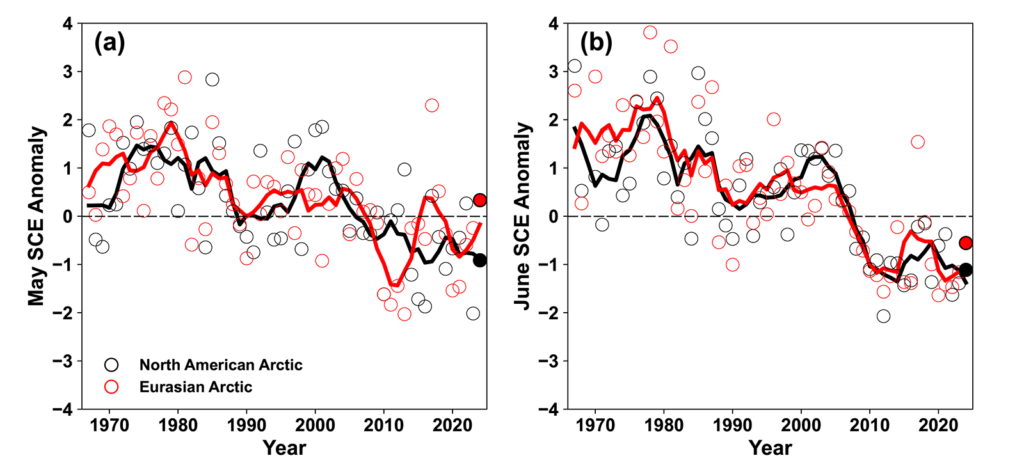
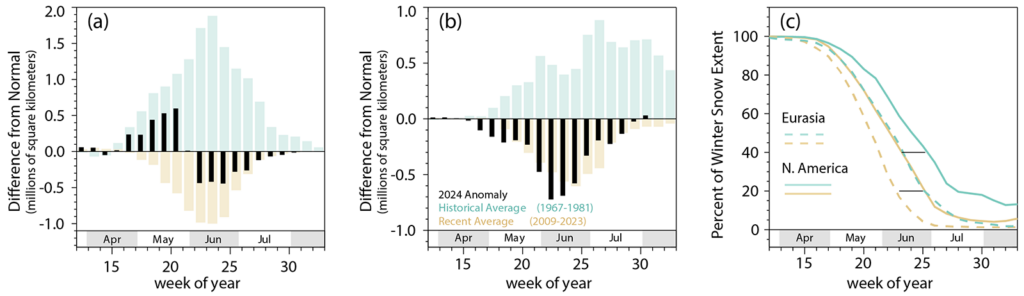
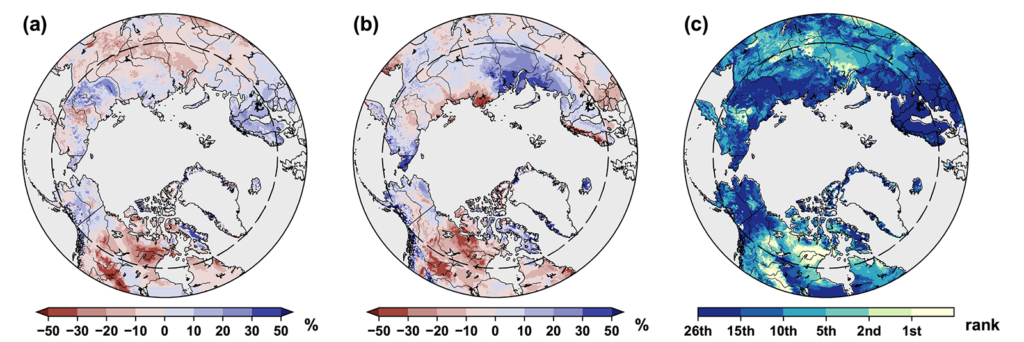
In contrast with Eurasia, North American Arctic SCE during 2024 was below normal throughout May and June (Figs. 1 and 2), with both months ranking 7th lowest in the 58-year record. Corresponding SCD anomalies indicate a combination of late onset and early spring melt that resulted in one of the shortest snow seasons of the last 26 years across portions of the Canadian Northwest Territories and mainland Nunavut (Fig. 3c).
Snow mass and snow water equivalent
Snow mass across the Arctic typically peaks annually during April, when snowfall has accumulated since the preceding autumn but before increasing temperatures during May and June lead to snow melt. Snow mass anomalies for April 2024 (Fig. 4) were above the 1991-2020 baseline across both the North American and Eurasian Arctic. The spatial patterns of monthly SWE (Fig. 5) illustrate how this accumulation varied regionally from just before peak (March) through to the end of the melt period (June). Positive SWE anomalies are apparent across broad portions of both continents in March and April. Strong reductions in the relative amount of SWE are apparent over the North American Arctic during May, while over Eurasia large regions of above-normal SWE still exist. By June the extent of Eurasian snow cover is much reduced, consistent with anomalies in Figs. 1 and 2b; however, the residual coverage between the Ob and Yenisei rivers and at the far eastern tip of the continent have above normal SWE consistent with the longer-than-normal melt season observed in those regions (Fig 3b). Over North America most of the Canadian Arctic Archipelago has below normal SWE during June except for Baffin Island.
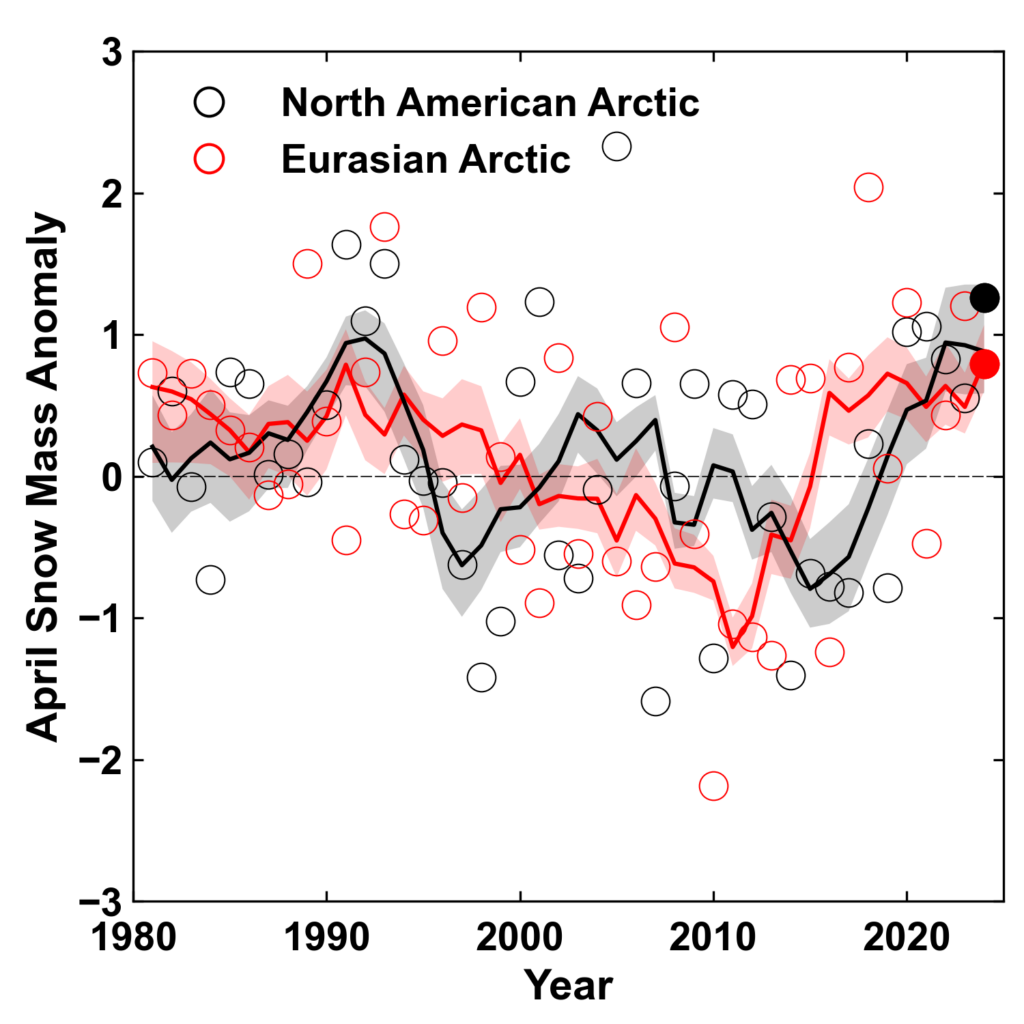
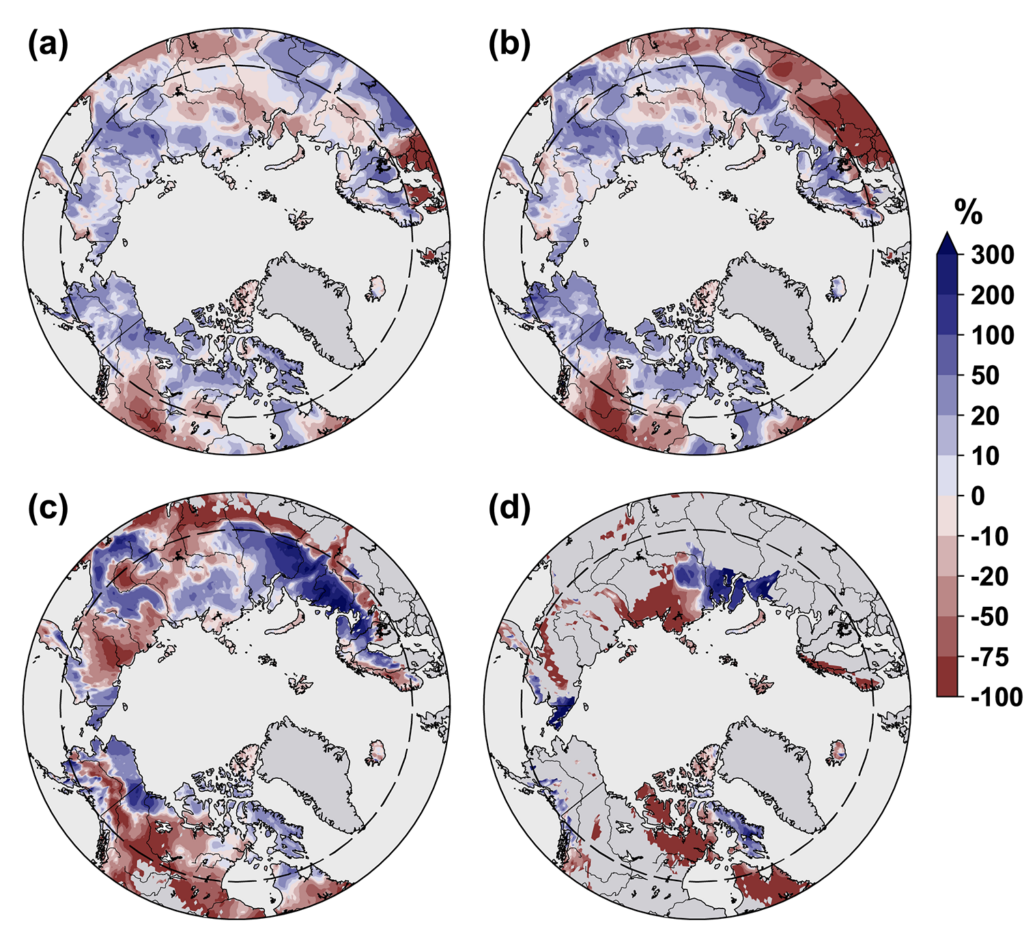
Summary and long-term changes to snowmelt timing
Snow accumulation during the 2023/24 winter was above average across both continents; however, the duration of the snow season contrasted strongly between them. Over North America warm spring temperatures resulted in snowmelt timing consistent with or even faster than what has typically been observed over the last 15 years (Fig 2a). Over broad portions of Eurasia, the above normal SWE persisted for most of May, keeping snow cover extent above levels typically seen for the last 15 years (Fig 2b). By June, however, Eurasian snow extent returned to values consistent with those seen over the past 15 years. Compared to historical conditions (1967-81), melt timing over the past 15 years (2009-23) has occurred 1-2 weeks earlier throughout May and June over both the Eurasian and North American Arctic.
Methods and data
SCE anomalies are derived from the NOAA weekly snow chart climate data record, which extends from 1967 to present (Robinson et al. 2012). Weekly anomalies of total areal snow cover over land for a given Arctic sector (North America or Eurasia, > 60° N) are computed relative to the 1991-2020 period. The same data are grouped by month and standardized (each observation differenced from the mean and divided by the standard deviation and thus unitless) to obtain standardized monthly anomalies of total areal snow cover relative to the same 1991-2020 period.
SCD fields are derived from the NOAA daily Interactive Multisensor Snow and Ice Mapping System (IMS) snow cover product (U.S. National Ice Center 2008). Anomalies in the total number of days with snow cover were computed separately for each half of the snow season: August 2023 to January 2024, referred to as “onset period,” and February 2024 to July 2024, referred to as “melt period.” IMS availability starts in 1998, so a 1998/99 to 2022/23 climatological period (25 years) is used (including information from August-December 1998 for snow onset). Anomalies for each season are presented as percent differences from the climatological number of snow-free days. Because the Arctic is generally always snow covered between November and April, Arctic region snow onset anomalies are indicative of conditions during September and October, while Arctic region snow melt anomalies are indicative of conditions during May and June.
Four SWE data sets were used to generate multi-data set SWE fields from March-June (inclusive) for the 1981-2024 period: (1) the European Space Agency Snow Climate Change Initiative (CCI) SWE version 2 product derived through a combination of satellite passive microwave brightness temperatures and climate station snow depth observations (Luojus et al. 2022); (2) the Modern-Era Retrospective Analysis for Research and Applications version 2 (MERRA-2, GMAO 2015) daily SWE fields; (3) SWE output from the ERA5-Land analysis (Muñoz Sabater 2019); and (4) the physical snowpack model Crocus (Decharme 2024) driven by ERA5 meteorological forcing. Limited availability of climate station snow data during May and June lowers the Snow CCI SWE product accuracy during these months; therefore, we only use it during March and April. An approach using gridded products is required because in situ observations alone are too sparse to capture snow conditions across the Arctic. We consider multiple data sets because averaging multiple SWE products has been shown to be more accurate than individual data sets when validated with in situ observations (Mortimer et al 2020). The ensemble-mean SWE field is used to calculate monthly SWE anomalies relative to the 1991-2020 period, which are presented as percent differences. For April, the SWE fields for each product are also aggregated across Arctic land regions (> 60° N) for both North American and Eurasian sectors to produce multiple estimates of April snow mass. These monthly snow mass values are used to calculate standardized anomalies relative to the 1991-2020 period for each data product. The standardized anomalies are then averaged to produce an ensemble-mean time series. Greenland is primarily covered by ice rather than snow and is not represented consistently among the SWE data products. For these reasons snow cover over the land mass is not added to SCE of either Arctic sector nor included in calculations of snow mass.
Acknowledgments
ERA5-Land data (Muñoz Sabater 2019) was downloaded from the Copernicus Climate Change Service (C3S) Climate Data Store (2024). Neither the European Commission nor ECMWF is responsible for any use of the Copernicus information or data it contains.
References
Brown, R., and Coauthors, 2017: Arctic terrestrial snow cover. Snow, Water, Ice and Permafrost in the Arctic (SWIPA) 2017. pp. 25-64. Arctic Monitoring and Assessment Programme (AMAP), Oslo, Norway.
Decharme, B., 2024: Crocus-ERA5 daily snow product over the Northern Hemisphere at 0.25° resolution (Version 2023), Zenodo, accessed 3 September 2024, https://doi.org/10.5281/zenodo.10943718.
GMAO (Global Modeling and Assimilation Office), 2015: MERRA-2tavg1_2d_lnd_Nx:2d, 1-Hourly, Time-Averaged, Single-Level, Assimilation, Land Surface Diagnostics V5.12.4, Goddard Earth Sciences Data and Information Services Center (GESDISC), accessed: 3 August 2024, https://doi.org/10.5067/RKPHT8KC1Y1T.
Luojus, K., and Coauthors, 2022: ESA Snow Climate Change Initiative (Snow_cci): Snow Water Equivalent (SWE) level 3C daily global climate research data package (CRDP) (1979-2020), version 2.0. NERC EDS Centre for Environmental Data Analysis, accessed: 3 September 2024, https://doi.org/10.5285/4647cc9ad3c044439d6c643208d3c494.
Meredith, M., and Coauthors, 2019: Polar Regions. IPCC Special Report on the Ocean and Cryosphere in a Changing Climate, H.-O. Pörtner, and co-editors, Cambridge University Press, Cambridge, UK and New York, NY, USA, 203-320, https://doi.org/10.1017/9781009157964.005.
Mortimer, C., L. Mudryk, C. Derksen, K. Luojus, R. Brown, R. Kelly, and M. Tedesco, 2020: Evaluation of long-term Northern Hemisphere snow water equivalent products. Cryosphere, 14, 1579-1594, https://doi.org/10.5194/tc-14-1579-2020.
Muñoz Sabater, J., 2019: ERA5-Land hourly data from 1950 to present. Copernicus Climate Change Service (C3S) Climate Data Store (CDS), accessed 3 September 2024, https://doi.org/10.24381/cds.e2161bac.
Robinson, D. A., T. W. Estilow, and NOAA CDR Program, 2012: NOAA Climate Data Record (CDR) of Northern Hemisphere (NH) Snow Cover Extent (SCE), Version 1 [r01]. NOAA National Centers for Environmental Information, accessed: 3 September 2024, https://doi.org/10.7289/V5N014G9.
U.S. National Ice Center, 2008: IMS Daily Northern Hemisphere Snow and Ice Analysis at 1 km, 4 km, and 24 km Resolutions, Version 1. Boulder, Colorado, USA. NSIDC: National Snow and Ice Data Center, accessed: 3 August 2024, https://doi.org/10.7265/N52R3PMC.
November 11, 2024