G. V. Frost1, U. S. Bhatt2, H. E. Epstein3, D. A. Walker4, M. K. Raynolds4, L. T. Berner5, J. W. Bjerke6, A. L. Breen2, B. C. Forbes7, S. J. Goetz5, C. M. Iversen8, M. J. Lara9, M. J. Macander1, G. K. Phoenix10, A. V. Rocha11, V. G. Salmon8, P. E. Thornton8, H. Tømmervik6, and S. D. Wullschleger8
1Alaska Biological Research, Inc., Fairbanks, AK, USA
2International Arctic Research Center, University of Alaska Fairbanks, Fairbanks, AK, USA
3Department of Environmental Sciences, University of Virginia, Charlottesville, VA, USA
4Institute of Arctic Biology, University of Alaska Fairbanks, Fairbanks, AK, USA
5School of Informatics, Computing, and Cyber Systems, Northern Arizona University, Flagstaff, AZ, USA
6Norwegian Institute for Nature Research, FRAM – High North Research Centre for Climate and the Environment, Tromsø, Norway
7Arctic Centre, University of Lapland, Rovaniemi, Finland
8Environmental Sciences Division and Climate Change Science Institute, Oak Ridge National Laboratory, Oak Ridge, TN, USA
9Department of Plant Biology and Department of Geography, University of Illinois at Urbana-Champaign, IL, USA
10Department of Animal and Plant Sciences, University of Sheffield, Sheffield, UK
11Department of Biological Sciences, University of Notre Dame, Notre Dame, IN, USA
Highlights
- The long-term satellite record (1982-2018) indicates “greening” across most Arctic tundra regions, especially Alaska’s North Slope, mainland Canada, and the Russian Far East, but trends are not homogeneous, and some regions instead exhibit no trend or “browning,” such as the Canadian Archipelago, southwestern Alaska, and parts of northwestern Siberia.
- In 2018, there were stark contrasts in tundra greenness by continent, with a sharp decline in greenness in North America but a modest increase in Eurasia.
- In North America, tundra productivity for the full growing season was the second lowest on record, concurrent with relatively cool spring and summer temperatures and late snowmelt in the Canadian Archipelago and Greenland.
Arctic lands and seas have experienced dramatic environmental and climatic changes in recent decades. These changes have been reflected in progressive increases in the aboveground quantity of live vegetation across most of the Arctic tundra biome—the treeless environment encircling most of the Arctic Ocean. This trend of increasing biomass is often referred to as “the greening of the Arctic.” Trends in tundra productivity, however, have not been uniform in direction or magnitude across the circumpolar region and there has been substantial variability from year to year (Bhatt et al. 2013, 2017; Park et al. 2016; National Academies of Sciences, Engineering, and Medicine 2019). Sources of spatial and temporal variability in tundra greenness arise from complex interactions among the vegetation, atmosphere, sea-ice, seasonal snow cover, ground (soils, permafrost, and topography), disturbance processes, and herbivores of the Arctic system.
Many of the changes being observed in tundra vegetation are producing a cascade of effects on the structure and function of Arctic ecosystems. For example, changes in the height and vigor of tundra plants impact the cycling of carbon and nutrients (Blume-Werry et al. 2019; Hewitt et al. 2019; Mörsdorf et al. 2019; Salmon et al. 2019; Treharne et al. 2019), as well as the exchange of energy between the atmosphere and permafrost soils (Wilcox et al. 2019). The latter has implications for permafrost stability and surface wetness which, coupled with changes in vegetation structure, can strongly alter habitat conditions for wildlife (Cray and Pollard 2018; Tape et al. 2018; Taylor et al. 2018; Ims et al. 2019; Kolari et al. 2019). Continued monitoring of circumpolar Arctic vegetation using Earth-observing satellites and field studies improves our understanding of these complex interactions and their impacts within, and beyond the Arctic.
Since 1982, a constellation of NOAA Earth-observing satellites has continually monitored the productivity of Arctic vegetation using a spectral metric termed the Normalized Difference Vegetation Index (NDVI), which is sensitive to the unique properties of photosynthetically-active vegetation in the Red and Near Infrared wavelengths. NDVI is highly correlated with the quantity of aboveground vegetation, or “greenness,” of Arctic tundra (Raynolds et al. 2012). The data reported here come from the Global Inventory Modeling and Mapping Studies 3g V1.1 dataset (GIMMS-3g); it is a biweekly, maximum-value composited dataset of the NDVI with a spatial resolution of 1/12° based on the Advanced Very High Resolution Radiometer (AVHRR) sensor (Pinzon and Tucker 2014). At the time of writing, the GIMMS-3g dataset was available only through the 2018 growing season. We use two metrics based on the NDVI: MaxNDVI and TI-NDVI. MaxNDVI is the peak NDVI value for the year and is related to the annual maximum biomass of aboveground vegetation that is reached in midsummer (typically late July or early August). TI (time-integrated) NDVI is the sum of the biweekly NDVI values for the growing season and is correlated with the total aboveground vegetation productivity. NDVI is generally highest near treeline and declines with increasing latitude. Values for North American tundra average lower than those for Eurasia, because a larger proportion of the North American Arctic lies in the High Arctic and was glaciated much more recently.
The GIMMS-3g record now spans 37 years (1982-2018) and indicates that both MaxNDVI and TI-NDVI have increased across most of the circumpolar Arctic tundra biome (Fig. 1a,b). Regions with the strongest greening include the Alaska’s North Slope, the Low Arctic (southern tundra subzones) of mainland Canada, and the Russian Far East. Tundra greenness appears to have declined, however, in western Alaska, the Canadian Archipelago, and parts of northwestern Siberia. Regional “hotspots” of greening and browning, evident as NDVI increases and decreases, respectively, are generally consistent between the two NDVI metrics, but decreases in TI-NDVI have been more widespread than decreases in MaxNDVI. In recent years, similar NDVI datasets have been compiled from other satellites and can be used to corroborate the GIMMS-3g record, albeit with a shorter period-of-record. For example, a recent analysis of the Landsat record indicates widespread increases in MaxNDVI from 1985 to 2018; greening was evident at 47% of sampling sites, but significant browning occurred at only 2% of sampling sites. The remaining 51% of sampling sites showed no deterministic trend over this period (L. Berner, personal communication).
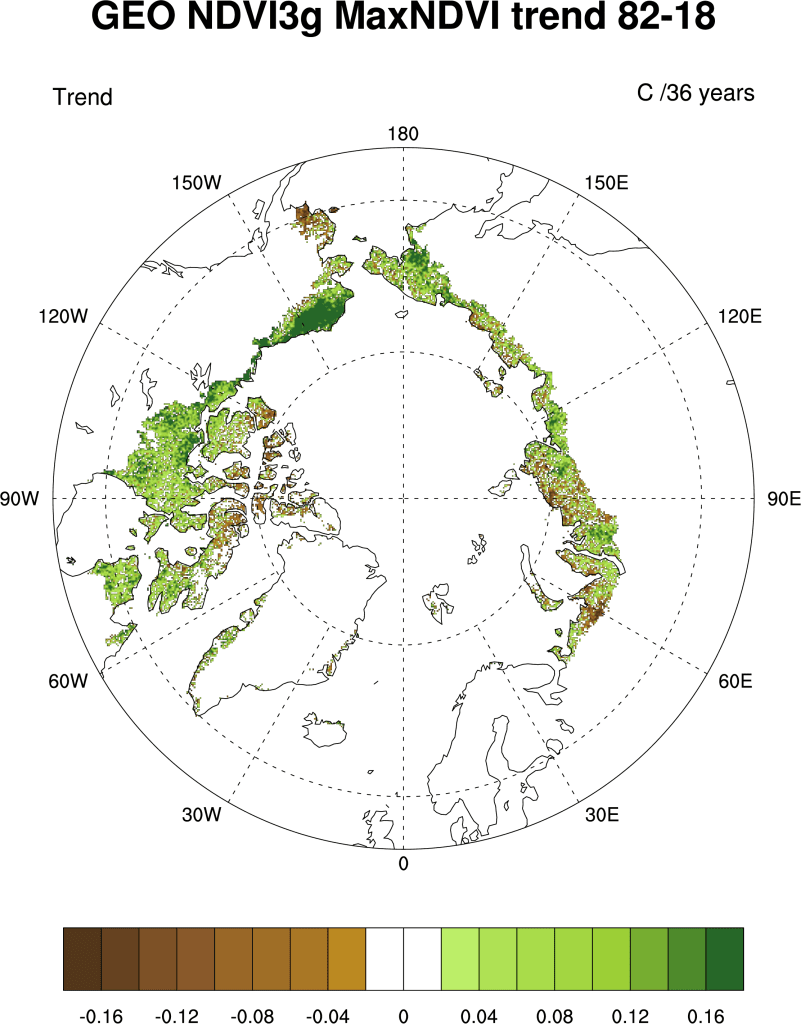
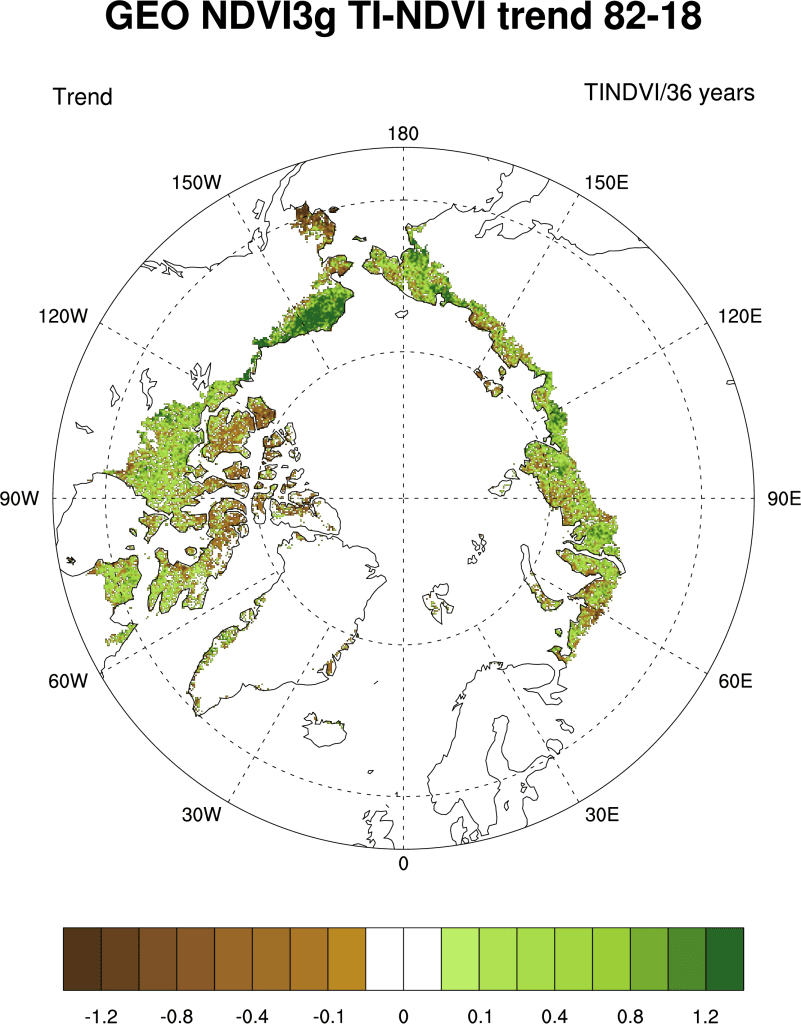
In 2018, growing season conditions and tundra productivity contrasted starkly between North America and Eurasia. NDVI in the Eurasian Arctic was similar to the previous year, but declined sharply in the North American Arctic (Fig. 2a,b), much of which experienced late snowmelt and relatively cool summer temperatures (Mudryk et al. 2018; Schmidt et al. 2019). Considering the circumpolar Arctic as a whole, NDVI declined for the second straight year; the decline was most obvious for TI-NDVI, for which the highest value in the record occurred in 2016. The 2018 TI-NDVI in North America fell well below the long-term median value and was the second lowest in the entire record behind 1992, when Arctic summer warmth was affected by atmospheric aerosols from the 1991 eruption of Mt. Pinatubo (Lucht 2002). TI-NDVI in North America declined 11.1% from 2017 to 2018, the largest single-year decline in the 37-year record. The year-to-year decline in MaxNDVI in North America was not as sharp (3.0%) and the 2018 value was near the long-term median. In the Eurasian Arctic, however, TI-NDVI increased slightly and MaxNDVI was steady; both values were above the long-term median.
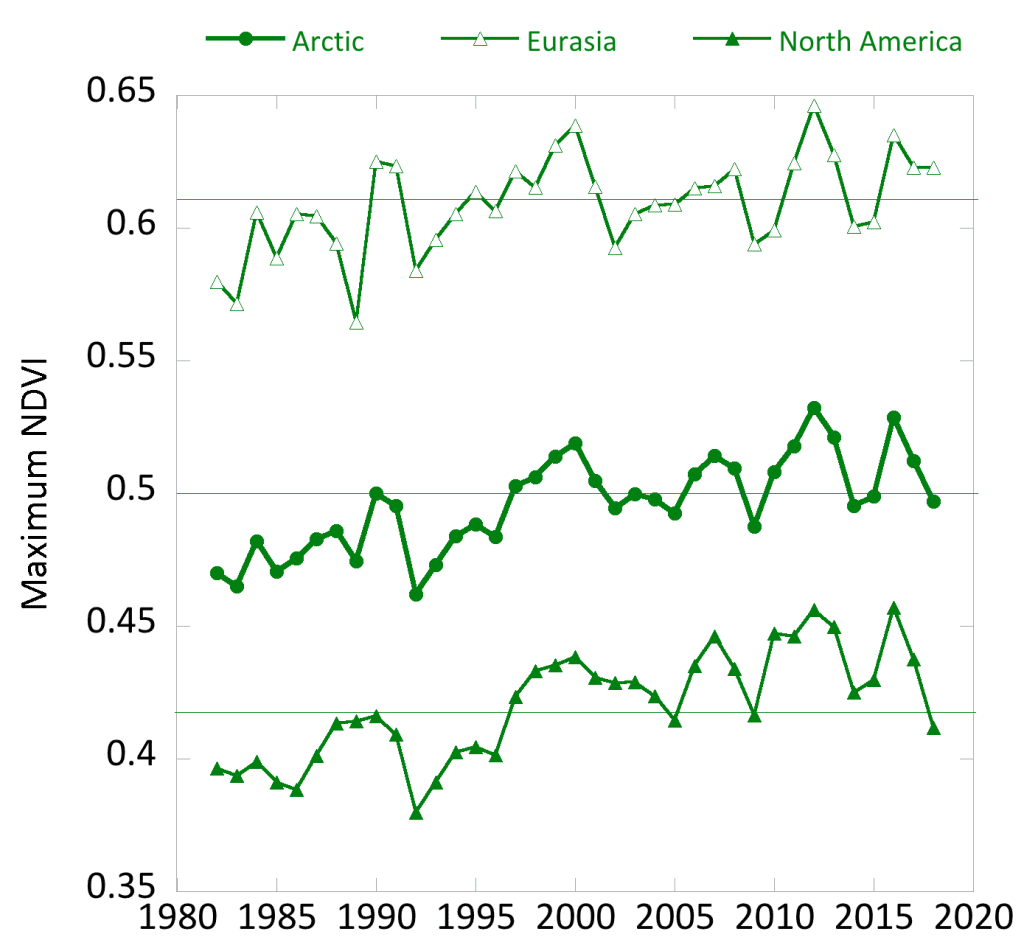
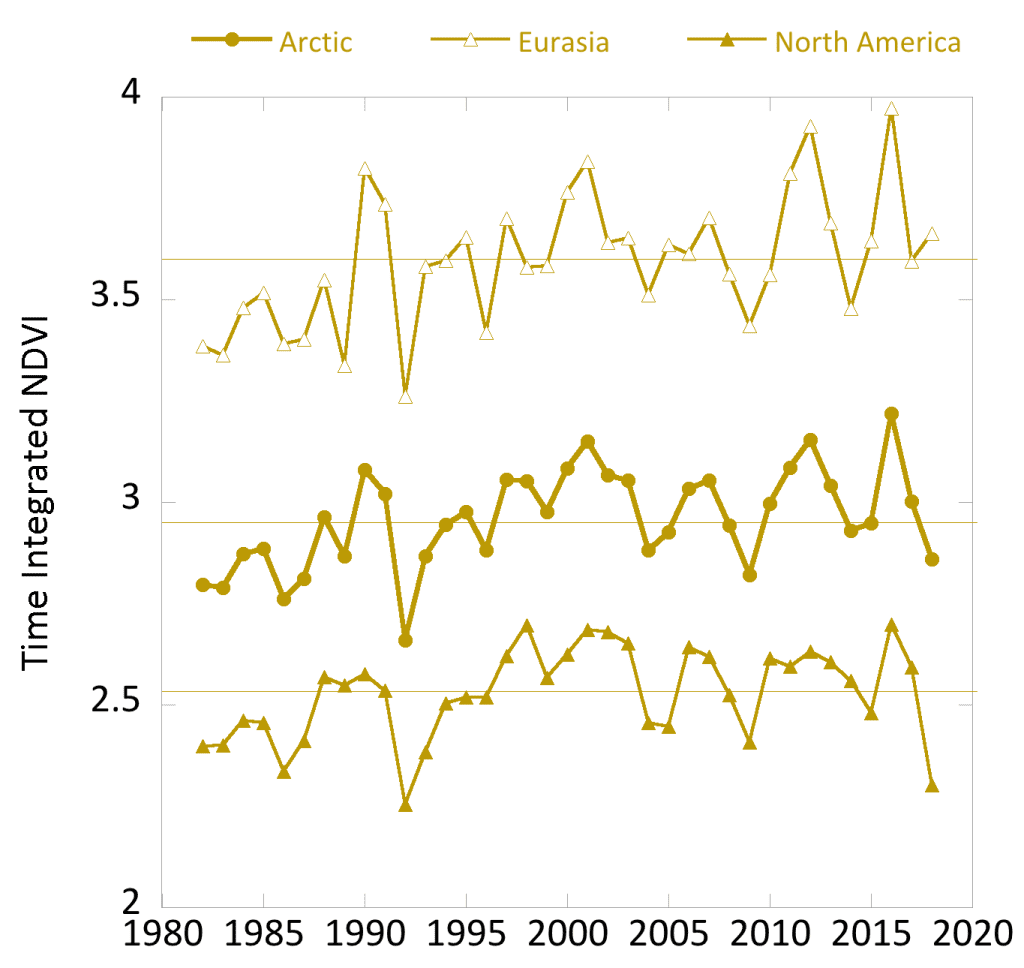
Within the 37-year record, MaxNDVI values for 2018 ranked 19th, 9th, and 25th for the circumpolar Arctic, Eurasian Arctic, and North American Arctic, respectively. TI-NDVI values ranked 31st, 11th, and 36th for the circumpolar Arctic, Eurasian Arctic, and North American Arctic respectively. Circumpolar measurements of summer warmth–a key control of NDVI–provide valuable context for understanding the strong contrasts in tundra productivity observed by continent in 2018. The AVHRR sensors that record NDVI values also record the Land Surface Temperature (LST). To evaluate temperature over the entire growing season, we summarize the LST observations as the Summer Warmth Index (SWI), the sum of mean monthly LST for months with mean temperatures above freezing (> 0°C). For the circumpolar Arctic region as a whole, SWI in 2018 was similar to the prior year and was the 6th highest in the record. In Eurasia, SWI increased sharply from the previous year and was the 2nd highest in the 37-year record, second only to 2016. In North America, however, the 2018 SWI declined sharply from 2017 and was the lowest recorded since 2009.
What are the drivers of Arctic vegetation change, and what types of change would be apparent to an observer on the ground? A growing number of long-term field studies have documented changes in vegetation and the physical environment that could account for the trends recorded by satellites (Jorgenson et al. 2015; Pattison et al. 2015; Myers-Smith et al. 2019). The productivity of tundra plants in a given year is influenced by many climatic and environmental factors, but increasing summer air temperature is widely acknowledged as the most influential driver of the long-term greening observed in many Arctic environments (Myers-Smith and Hik 2018; Pastick et al. 2019; see essay Surface Air Temperature). Arctic shrub expansion is a well-recognized tundra response to summer warming, particularly in moist habitats of the Low Arctic (Elmendorf et al. 2012; Myers-Smith et al. 2015; Salmon et al. 2019). On the other hand, recent reports show that increased temperatures can have disruptive effects on the phenology and growth of tundra plants (Assmann et al. 2019; Prevéy et al. 2019).
Tundra “browning” has been observed after extreme weather, such as winter thaw events (followed by abrupt cooling and desiccation) and icing (e.g., rain-on-snow); recent work in Norway suggests that such events reduce the capacity of the vegetation to sequester carbon (Treharne et al. 2019). Changes in precipitation, permafrost conditions, and soil moisture also influence tundra vegetation dynamics and are linked to the effects of air temperature changes (Lara et al. 2018; Kemppinen et al. 2019). Increased winter snow cover can also alter vegetation structure and function, in some cases countering the impact of warming (Addis and Bret-Harte 2019; Cooper et al. 2019). Ecological disturbances such as tundra fire can also cause abrupt changes in greenness over large areas (French et al. 2016); at local scales, permafrost thaw and herbivory can create “hotspots” of greening and browning (Lara et al. 2018; Pastick et al. 2019). Disparate vegetation responses to temperature and precipitation are also evident when comparing plant communities along gradients of latitude (Miles et al. 2019), elevation (Verbyla and Kurkowski 2019), soil moisture (Rocha et al. 2018), and human land-use (Chen et al. 2019; Tømmervik et al. 2019). These findings highlight the importance of understanding indirect responses to climate warming that occur at local and regional scales when interpreting Arctic NDVI trends.
References
Addis, C. E., and M. S. Bret-Harte, 2019: The importance of secondary growth to plant responses to snow in the arctic. Funct. Ecol., 33, 1050-1066.
Assmann, J. J., I. H. Myers-Smith, A. B. Phillimore, A. D. Bjorkman, R. E. Ennos, J. S. Prevéy, G. H. R. Henry, N. M. Schmidt, and R. D. Hollister, 2019: Local snow melt and temperature-but not regional sea ice-explain variation in spring phenology in coastal Arctic tundra. Glob. Change Biol., 25, 2258–2274, https://doi.org/10.1111/gcb.14639.
Bhatt, U. S., D. A. Walker, M. K. Raynolds, P. A. Bieniek, H. E. Epstein, J. C. Comiso, J. E. Pinzon, C. J. Tucker, M. Steele, W. Ermold, and J. Zhang, 2017: Changing seasonality of panarctic tundra vegetation in relationship to climatic variables. Environ. Res. Lett., 12, 055003.
Bhatt, U., D. Walker, M. Raynolds, P. Bieniek, H. Epstein, J. Comiso, J. Pinzon, C. Tucker, and I. Polyakov, 2013: Recent declines in warming and vegetation greening trends over Pan-Arctic tundra. Remote Sens., 5, 4229-4254.
Blume-Werry, G., A. Milbau, L. M. Teuber, M. Johansson, and E. Dorrepaal, 2019: Dwelling in the deep – strongly increased root growth and rooting depth enhance plant interactions with thawing permafrost soil. New Phytol., 223, 1328-1339.
Chen, C., T. Park, X. Wang, S. Piao, B. Xu, R. K. Chaturvedi, R. Fuchs, V. Brovkin, P. Ciais, R. Fensholt, H. Tømmervik, G. Bala, Z. Zhu, R. R. Nemani, and R. B. Myneni, 2019: China and India lead in greening of the world through land-use management. Nat. Sustain., 2, 122-129.
Cooper, E. J., C. J. Little, A. K. Pilsbacher, and M. A. Mörsdorf, 2019: Disappearing green: Shrubs decline and bryophytes increase with nine years of increased snow accumulation in the High Arctic. J. Veg. Sci., 30, 857-867.
Cray, H. A., and W. H. Pollard, 2018: Use of stabilized thaw slumps by Arctic birds and mammals: evidence from Herschel Island, Yukon. Can. Field Nat., 132, 279-284.
Elmendorf, S. C., G. H. R. Henry, R. D. Hollister, R. G. Björk, N. Boulanger-Lapointe, E. J. Cooper, J. H. C. Cornelissen, T. A. Day, E. Dorrepaal, T. G. Elumeeva, M. Gill, W. A. Gould, J. Harte, D. S. Hik, A. Hofgaard, D. R. Johnson, J. F. Johnstone, I. S. Jónsdóttir, J. C. Jorgenson, K. Klanderud, J. A. Klein, S. Koh, G. Kudo, M. Lara, E. Lévesque, B. Magnússon, J. L. May, J. A. Mercado-Díaz, A. Michelsen, U. Molau, I. H. Myers-Smith, S. F. Oberbauer, V. G. Onipchenko, C. Rixen, N. Martin Schmidt, G. R. Shaver, M. J. Spasojevic, Þ. E. Þórhallsdóttir, A. Tolvanen, T. Troxler, C. E. Tweedie, S. Villareal, C. -H. Wahren, X. Walker, P. J. Webber, J. M. Welker, and S. Wipf, 2012: Plot-scale evidence of tundra vegetation change and links to recent summer warming. Nat. Climate Change, 2, 453-457.
French, N. H. F., M. A. Whitley, and L. K. Jenkins, 2016: Fire disturbance effects on land surface albedo in Alaskan tundra. J. Geophys.Res.-Biogeosci., 12, 841-854.
Hewitt, R. E., D. L. Taylor, H. Genet, A. D. McGuire, and M. C. Mack, 2019: Below-ground plant traits influence tundra plant acquisition of newly thawed permafrost nitrogen. J. Ecol., 107, 950-962.
Ims, R. A., J. -A. Henden, M. A. Strømeng, A. V. Thingnes, M. J. Garmo, and J. U. Jepsen, 2019: Arctic greening and bird nest predation risk across tundra ecotones. Nat. Clim. Change, 9, 607-610.
Jorgenson, J. C., M. K. Raynolds, J. H. Reynolds, and A. -M. Benson, 2015: Twenty-five year record of changes in plant cover on tundra of northeastern Alaska. Arct. Antarct. Alp. Res., 47, 785-806.
Kemppinen, J., P. Niittynen, J. Aalto, P. C. le Roux, and M. Luoto, 2019: Water as a resource, stress and disturbance shaping tundra vegetation. Oikos, 128, 811-822.
Kolari, T. H. M., T. Kumpula, M. Verdonen, B. C. Forbes, and T. Tahvanainen, 2019: Reindeer grazing controls willows but has only minor effects on plant communities in Fennoscandian oroarctic mires. Arct. Antarct. Alp. Res., 51, 506-520.
Lara, M. J., I. Nitze, G. Grosse, P. Martin, and A. D. McGuire, 2018: Reduced arctic tundra productivity linked with landform and climate change interactions. Sci. Rep.-UK, 8, 2345.
Lucht, W., 2002: Climatic control of the high-latitude vegetation greening trend and Pinatubo effect. Science, 296, 1687-1689.
Miles, M. W., V. V. Miles, and I. Esau, 2019: Varying climate response across the tundra, forest-tundra and boreal forest biomes in northern West Siberia. Environ. Res. Lett., 14, 075008.
Mörsdorf, M. A., N. S. Baggesen, N. G. Yoccoz, A. Michelsen, B. Elberling, P. L. Ambus, and E. J. Cooper, 2019: Deepened winter snow significantly influences the availability and forms of nitrogen taken up by plants in High Arctic tundra. Soil Biol. Biochem., 135, 222-234.
Mudryk, L., R. Brown, C. Derksen, K. Luojus, B. Decharme, and S. Helfrich, 2018: Terrestrial snow cover. Arctic Report Card 2018, E. Osborne, J. Richter-Menge, and M. Jeffries, Eds., https://www.arctic.noaa.gov/Report-Card.
Myers-Smith, I. H., S. C. Elmendorf, P. S. A. Beck, M. Wilmking, M. Hallinger, D. Blok, K. D. Tape, S. A. Rayback, M. Macias-Fauria, B. C. Forbes, J. D. M. Speed, N. Boulanger-Lapointe, C. Rixen, E. Lévesque, N. M. Schmidt, C. Baittinger, A. J. Trant, L. Hermanutz, L. S. Collier, M. A. Dawes, T. C. Lantz, S. Weijers, R. H. Jørgensen, A. Buchwal, A. Buras, A. T. Naito, V. Ravolainen, G. Schaepman-Strub, J. A. Wheeler, S. Wipf, K. C. Guay, D. S. Hik, and M. Vellend, 2015: Climate sensitivity of shrub growth across the tundra biome. Nat. Clim. Change, 5, 887-891.
Myers-Smith, I. H., M. M. Grabowski, H. J. D. Thomas, S. Angers-Blondin, G. N. Daskalova, A. D. Bjorkman, A. M. Cunliffe, J. J. Assmann, J. S. Boyle, E. McLeod, S. McLeod, R. Joe, P. Lennie, D. Arey, R. R. Gordon, and C. D. Eckert, 2019: Eighteen years of ecological monitoring reveals multiple lines of evidence for tundra vegetation change. Ecol. Monogr., 89, e01351.
Myers-Smith, I. H., and D. S. Hik, 2018: Climate warming as a driver of tundra shrubline advance. R. Aerts, Ed. J. Ecol., 106, 547-560.
National Academies of Sciences, Engineering, and Medicine, 2019: Understanding northern latitude vegetation greening and browning: proceedings of a workshop. A. Melvin, Ed. The National Academies Press, Washington, DC.
Park, T., S. Ganguly, H. Tømmervik, E. S. Euskirchen, K. -A. Høgda, S. R. Karlsen, V. Brovkin, R. R. Nemani, and R. B. Myneni, 2016: Changes in growing season duration and productivity of northern vegetation inferred from long-term remote sensing data. Environ. Res. Lett., 11, 084001.
Pastick, N. J., M. T. Jorgenson, S. J. Goetz, B. M. Jones, B. K. Wylie, B. J. Minsley, H. Genet, J. F. Knight, D. K. Swanson, and J. C. Jorgenson, 2019: Spatiotemporal remote sensing of ecosystem change and causation across Alaska. Glob. Change Biol., 25, 1171-1189.
Pattison, R. R., J. C. Jorgenson, M. K. Raynolds, and J. M. Welker, 2015: Trends in NDVI and tundra community composition in the arctic of NE Alaska between 1984 and 2009. Ecosystems, 18, 707-719.
Pinzon, J., and C. Tucker, 2014: A Non-stationary 1981-2012 AVHRR NDVI3g time series. Remote Sens., 6, 6929-6960.
Prevéy, J. S., C. Rixen, N. Rüger, T. T. Høye, A. D. Bjorkman, I. H. Myers-Smith, S. C. Elmendorf, I. W. Ashton, N. Cannone, C. L. Chisholm, K. Clark, E. J. Cooper, B. Elberling, A. M. Fosaa, G. H. R. Henry, R. D. Hollister, I. S. Jónsdóttir, K. Klanderud, C. W. Kopp, E. Lévesque, M. Mauritz, U. Molau, S. M. Natali, Steven. F. Oberbauer, Z. A. Panchen, E. Post, S. B. Rumpf, N. M. Schmidt, E. Schuur, P. R. Semenchuk, J. G. Smith, K. N. Suding, Ø. Totland, T. Troxler, S. Venn, C. -H. Wahren, J. M. Welker, and S. Wipf, 2019: Warming shortens flowering seasons of tundra plant communities. Nat. Ecol. Evol., 3, 45-52.
Raynolds, M. K., D. A. Walker, H. E. Epstein, J. E. Pinzon, and C. J. Tucker, 2012: A new estimate of tundra-biome phytomass from trans-Arctic field data and AVHRR NDVI. Remote Sens. Lett., 3, 403-411.
Rocha, A. V., B. Blakely, Y. Jiang, K. S. Wright, and S. R. Curasi, 2018: Is arctic greening consistent with the ecology of tundra? Lessons from an ecologically informed mass balance model. Environ. Res. Lett., 13, 125007.
Salmon, V. G., A. L. Breen, J. Kumar, M. J. Lara, P. E. Thornton, S. D. Wullschleger, and C. M. Iversen, 2019: Alder distribution and expansion across a tundra hillslope: implications for local N cycling. Front. Plant Sci., 10, 1099, https://doi.org/10.3389/fpls.2019.01099.
Schmidt, N. M., J. Reneerkens, J. H. Christensen, M. Olesen, and T. Roslin, 2019: An ecosystem-wide reproductive failure with more snow in the Arctic. PLOS Biol., 17, e3000392.
Tape, K. D., B. M. Jones, C. D. Arp, I. Nitze, and G. Grosse, 2018: Tundra be dammed: Beaver colonization of the Arctic. Glob. Change Biol., 24, 4478-4488.
Taylor, A. R., R. B. Lanctot, and R. T. Holmes, 2018: An evaluation of 60 years of shorebird response to environmental change at Utqiaġvik (Barrow), Alaska. Trends and Traditions: Avifaunal Change in Western North America, W. D. Shuford, R. E. Gill, and C. M. Handel, Eds., Western Field Ornithologists, 312-330, accessed 24 September 2019, http://www.wfopublications.org/Avifaunal_Change/Taylor.
Tømmervik, H., J. W. Bjerke, T. Park, F. Hanssen, and R. B. Myneni, 2019: Legacies of historical exploitation of natural resources are more important than summer warming for recent biomass increases in a boreal-arctic transition region. Ecosystems, 22, 1512, https://doi.org/10.1007/s10021-019-00352-2.
Treharne, R., J. W. Bjerke, H. Tømmervik, L. Stendardi, and G. K. Phoenix, 2019: Arctic browning: Impacts of extreme climatic events on heathland ecosystem CO2 fluxes. Glob. Change Biol., 25, 489-503.
Verbyla, D., and T. A. Kurkowski, 2019: NDVI-Climate relationships in high-latitude mountains of Alaska and Yukon Territory. Arct. Antarct. Alp. Res., 51, 397-411.
Wilcox, E. J., D. Keim, T. de Jong, B. Walker, O. Sonnentag, A. E. Sniderhan, P. Mann, and P. Marsh, 2019: Tundra shrub expansion may amplify permafrost thaw by advancing snowmelt timing. Arct. Sci., https://doi.org/10.1139/as-2018-0028.
November 19, 2019